Analyzing Key Chemical Products and Their Impact
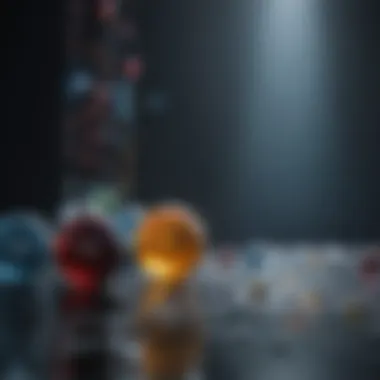
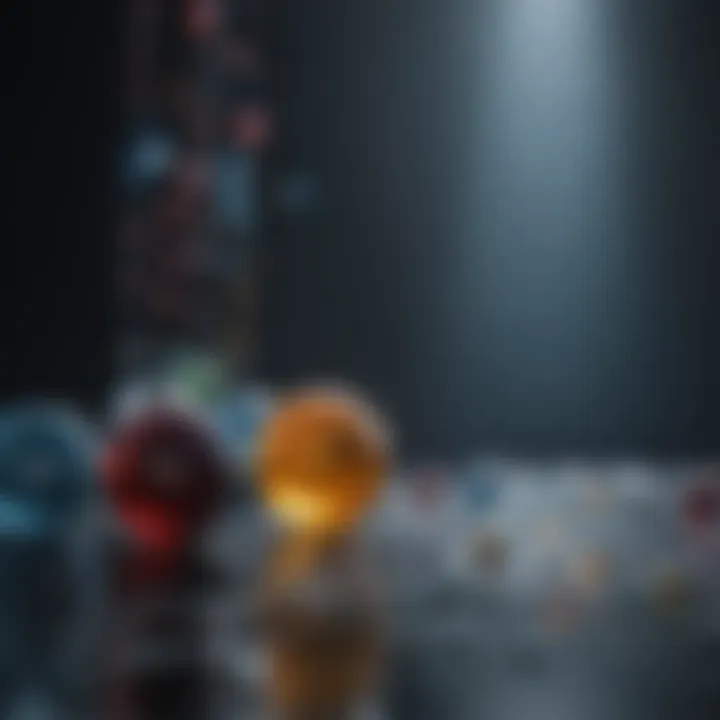
Intro
Chemistry is often thought of as a collection of formulas and reactions, but its impact stretches far beyond the laboratory. The products generated through chemical processes are woven into the fabric of our daily lives, enhancing industries from healthcare to agriculture. To appreciate the complexity and significance of these products, one must grasp the foundational concepts that govern their existence and application.
This exploration of chemical products aims to bridge the gap between theoretical knowledge and practical implications. We’ll peel back the layers on how these substances are classified, synthesized, and ultimately utilized. In doing so, we uncover the driving forces of innovation and the challenges facing modern society as it navigates through an ever-evolving chemical landscape.
Classification of Chemical Products
The classification of chemical products serves as the backbone of chemistry itself. It allows scientists, educators, and practitioners to communicate effectively across various fields by numerous categories and properties. Understanding this classification is crucial for everything from research and development to practical applications in everyday life. It helps to not only streamline chemical production but also assists in ensuring safety and regulatory compliance, which are vital in today’s industrial landscape.
Chemical products are fundamentally split into two branches: organic and inorganic compounds. Each of these categories holds unique characteristics that determine their use in different areas like pharmaceuticals, agriculture, and materials science. By grasping these classifications, professionals can determine the right substances for their specific needs, ultimately contributing to advancements in technology and health.
Organic Compounds
Definition and Characteristics
Organic compounds are primarily carbon-based structures that often include hydrogen, nitrogen, oxygen, and other elements. They typically exhibit a distinctive property known as covalent bonding, where atoms share electron pairs. One of the notable characteristics of organic compounds is that they can form complex structures, allowing for a vast array of chemical behaviors and characteristics.
This versatility makes organic compounds a compelling choice for this analysis as they are the foundation of life on Earth. They are crucial in many fields, such as biochemistry and pharmaceuticals, because they engage in reactions that sustain biological processes. The flexibility of their structure leads to an extensive variety of products, from simple alcohols to intricate polymers. However, one needs to consider the environmental impact of some organic products, which can be drawbacks in certain applications.
Examples of Organic Products
When examining examples of organic products, one cannot overlook familiar items like ethanol, found in beverages, or glucose, a fundamental sugar utilized by living organisms. Other unique examples include medicinal compounds like aspirin or antibiotics. These products not only showcase organic chemistry's diversity but also highlight its relevance in daily life.
The beauty of these organic products lies in their ability to blend utility and complexity. They can be synthesized through various methods, but understanding their origins is crucial. Not all organic products come without challenges; some may present toxicity or stability issues, necessitating careful handling practices in both household and industrial settings.
Applications in Daily Life
The application of organic compounds is staggering in daily life. From the food we eat to the medicines we take, these compounds have directly impacted our health and lifestyle. For instance, many vitamins and supplements used in nutritional products are organic compounds. Moreover, organic chemistry plays a significant role in developing materials for clothing and packaging.
Highlighting their key characteristic of versatility, organic products can be seen in everything from paints to lubricants, making them invaluable. However, one must be aware of the limitations; some organic compounds can be harmful to health and the environment, compelling a push for more sustainable practices in their production and use.
Inorganic Compounds
Categories of Inorganic Compounds
Inorganic compounds encompass a broader category that typically does not include carbon-hydrogen bonds. They can be categorized into several groups, such as salts, metals, and minerals. Each category serves a specific purpose and has varying properties that contribute to their uses in various industries.
This classification is beneficial in this discussion because inorganic compounds often have crucial roles in chemistry and material sciences. For instance, metals such as iron and aluminum are essential for construction, while salts play numerous roles in chemical processes. The unique features of inorganic compounds, such as their generally higher melting points and electrical conductivity, make them indispensable in various applications, though their toxicity can be a potential disadvantage.
Common Inorganic Products
Common inorganic products include sodium chloride (table salt), silica (used in glass), and sulfuric acid. Each product serves unique yet fundamental roles in both laboratory settings and daily utilities. For example, sodium chloride is not only a seasoning but also a preservative and an essential nutrient.
Their practicality cannot be overstated; however, one must remain cautious, as certain inorganic products can corrode materials or pose health risks if mishandled. Recognizing safe handling and storage practices is vital in utilizing these chemical products effectively.
Relevance in Industrial Applications
The relevance of inorganic compounds in industry is tremendous. Industries like construction, manufacturing, and electronics rely heavily on various inorganic products. For example, metals are pervasive in the build of infrastructure, while ceramics and glass products are essential in consumer goods.
Their key characteristic of stability and durability is particularly beneficial. Whether it's the use of silica in electronics or the application of metals in machinery, the impact of inorganic compounds is irrefutable. Nonetheless, some applications can raise environmental concerns, sparking ongoing research into more sustainable practices within the production and utilization of these materials.
Synthesis of Chemical Products
Synthesis of chemical products stands at the very heart of the chemical industry. Without synthesis, compounds wouldn't be available to fuel advancements in healthcare, agriculture, and myriad other industries. It's like a chef crafting a fine dish from raw ingredients; the outcome heavily relies on the methods and precision employed. In this sense, synthesis not only ensures the availability of products but also allows for innovation, enabling scientists and engineers to create compounds tailored to specific applications.
Moreover, understanding synthesis is pivotal. It opens a window into how substances interact, how they can be manipulated, and ultimately, how they can better society in various ways. The choice between traditional methods and modern synthetic approaches can significantly affect efficiency, sustainability, and product efficacy.
Traditional Methods
Overview of Historical Techniques
Traditional methods of chemical synthesis have been the backbone of the chemistry field for centuries. Techniques such as distillation, crystallization, and evaporation are some examples that have stood the test of time. These methods are valued not only for their historical significance but also for their reliability. They often yield pure products with reasonable efficiency.
One of the key characteristics of these historical techniques is their adaptability. For instance, distillation can be used to separate mixtures based on boiling points, providing a clear path to isolating desired compounds from complex blends. This makes traditional methods a cornerstone for many laboratories, emphasizing their long-term profitability and global acceptance.
However, while traditional methods are beneficial in certain contexts, they can present challenges. For example, many of these techniques often require large amounts of energy or may produce undesired waste during processes. Hence, while they have a unique appeal for simplicity and cost-effectiveness, they may not always align with modern-day sustainability goals.
Limitations of Traditional Synthesis
Despite their historical value, traditional synthesis methods come with their own sets of limitations. For one, they often lack the ability to produce products with high specificity. This can result in by-products that could complicate purification processes and reduce efficiency — a major concern in today’s fast-paced industrial climate.
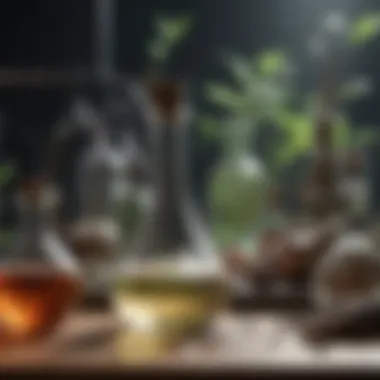
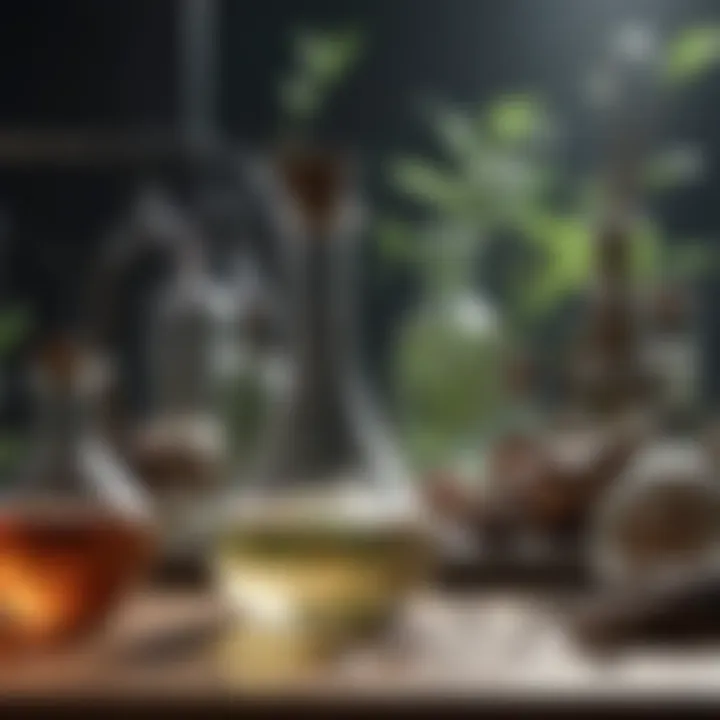
A defining aspect of these limitations is their scalability. While a lab can easily employ traditional methods for small batches, scaling these processes to meet larger demands can be a significant hurdle. Plus, many of these techniques do not reflect the stringent safety and environmental standards expected in many modern industries today. Therefore, traditional methods, while they possess intrinsic merit, often require a balancing act to modern requirements that call for eco-friendliness and minimized waste.
Modern Synthetic Approaches
Green Chemistry Principles
The landscape of chemical synthesis has evolved tremendously with the introduction of green chemistry principles. This modern approach emphasizes sustainability and the reduction of hazardous substances in chemical processes. By embracing these principles, scientists can design processes that are more efficient while prioritising safety and environmental conservation.
Key characteristics of green chemistry include its focus on minimizing waste and choosing solvents that are less harmful. This not only contributes positively to public health but also enhances the overall reputation of the chemical industry. Such attributes make green chemistry a compelling choice for contemporary syntheses and a focal point in educational discussions about responsible chemistry.
Nevertheless, implementing green chemistry can pose challenges. Approaches that are eco-friendly may require investment in new technologies or methods, which can deter larger companies from diversifying their processes. Yet, the long-term benefits — including enhanced safety and potential cost savings — often outweigh these initial obstacles.
Advancements in Catalysis
Advancements in catalysis have been instrumental in shaping modern synthetic approaches. Catalysis can dramatically enhance the speed of chemical reactions, making it a vital component of effective synthesis. Recent innovations in this field have brought forth new catalysts that operate under milder conditions, thereby expanding the potential of chemical reactions.
One remarkable feature of these advancements is their ability to streamline production processes, which can bolster efficiency significantly. By accelerating reactions and improving yields, newer catalysts reduce the overall consumption of materials and energy. This aligned drive toward efficiency makes these innovations not just beneficial but essential for modern chemistry.
However, with advantages come disadvantages. Some new catalysts may require complex preparation or sourcing of rare materials, which could lead to challenges in terms of cost and availability. Balancing the benefits of advanced catalysis with practical considerations is essential for its continued success in industrial settings.
Use of Biotechnology in Synthesis
Biotechnology has become a significant game-changer in the synthesis landscape. By harnessing living organisms or biological systems, scientists can create products in a manner that is often more efficient and environmentally friendly. This method can involve everything from fermentation in pharmaceuticals to using engineered bacteria to produce biofuels.
The primary characteristic of biotechnology that makes it particularly appealing is its ability to achieve specific transformations that may be challenging through traditional synthetic methods. For instance, biocatalysts can perform complex reactions under mild conditions, decreasing energy demands while enhancing product yields.
Nonetheless, the integration of biotechnology in synthesis isn't without pitfalls. Concerns regarding regulatory approval and public acceptance can slow advancements. Furthermore, technical challenges in scaling biotechnological processes need careful navigation. Still, the potential benefits of this approach can be tremendously influential in deciding how we approach chemical synthesis as we forge ahead into the future.
Whether through traditional systems or modern methods, the synthesis of chemical products represents a crucial area that binds together innovation, sustainability, and practical application across countless industries.
Application of Chemical Products
The application of chemical products is a cornerstone in bridging the theoretical aspects of chemistry with practical impacts on everyday life. Whether in medicine, agriculture, or materials science, chemical products play vital roles that often go unnoticed. Their importance is multifaceted, touching areas such as public health, food security, and innovation in technology. Understanding how these products are applied not only informs those in the chemistry field but also educates the general populace about implications for society.
In every aspect of application, from pharmaceuticals addressing chronic diseases, to fertilizers boosting agricultural output, the significance cannot be overstated. Each chemical product embodies a complex interplay of properties that contribute to specific needs, such as efficiency, safety, or sustainability.
"Chemistry does not merely reign over labs and textbooks; its echoes resonate in our healthcare systems and fields of grain."
Impact on Pharmaceuticals
Types of Pharmaceutical Products
Pharmaceutical products are as varied as the ailments they aim to treat. They could range from small-molecule drugs, like aspirin, to large biologics such as monoclonal antibodies used in cancer therapies. Each type possesses unique characteristics shaped by their molecular structures and intended uses.
One notable characteristic is the specificity of drug action. This is paramount, especially for conditions that require precise targeting to minimize side effects while maximizing therapeutic effects. The growing emphasis on personalized medicine underlines the shift toward developing specific types of pharmaceutical products, tailored to individual patient profiles.
A distinct feature of pharmaceutical products is their rigorous testing processes—from Discovery to clinical trials—ensuring they meet the highest safety and efficacy standards. However, this often results in lengthy development timelines, potentially delaying access to life-saving treatments.
Role of Chemistry in Drug Development
Chemistry is the bedrock upon which drug development stands, underpinning every step from design to market. The synthesis of new compounds heavily relies on the principles of chemistry; it involves a deep understanding of reaction mechanisms and molecular interactions.
A key characteristic of this process is the interdisciplinary approach, combining insights from biochemistry, pharmacology, and material science. This integration fosters innovation in developing drugs that can address previously untreatable conditions.
A unique aspect of chemistry's role in drug development is its reliance on computational methods, which can predict how different compounds will interact within the body. This efficiency leads to faster discovery times and a reduction in resource expenditure related to trial-and-error. However, reliance on simulations can also mislead, as not all predictions correlate with real-world experimentation.
Agricultural Chemicals
Pesticides and Herbicides
Agricultural chemicals, particularly pesticides and herbicides, are employed to combat unwanted pests and weeds, ensuring higher crop yields. The aspect of crop protection is essential for food security, and these chemicals often save farmers from substantial crop losses.
The effectiveness of pesticides lies in their specificity. In an ideal world, they only affect the target pests, preserving beneficial insects and wider biodiversity. Unfortunately, the reality sometimes diverges, leading to debates about environmental impact and the development of pesticide resistance among target populations.
A significant consideration here is that while they can increase production efficiency, over-reliance on pesticides can lead to ecosystem imbalances. This is a delicate tightrope that farmers must walk, balancing immediate agricultural needs with long-term environmental sustainability.
Fertilizers and Their Chemical Composition
Fertilizers contribute directly to enhancing soil fertility and plant growth. Their chemical composition, often rich in nitrogen, phosphorus, and potassium, addresses nutrient deficiencies that can impede agricultural productivity.
These compounds are often synthetic, enabling precise nutrient delivery tailored to specific crop needs. This targeted application is beneficial for maximizing yields, especially in intensive farming operations. However, the unintended consequences of chemical runoff into waterways highlight the pressing need for more responsible application practices.
A crucial feature of fertilizers is their dual role in both stimulating growth and, if misapplied, causing environmental harm. Thus, education around proper usage is becoming part and parcel of modern agricultural practices.
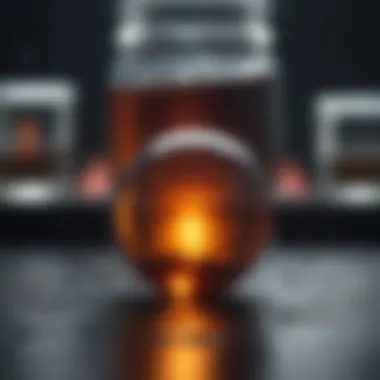
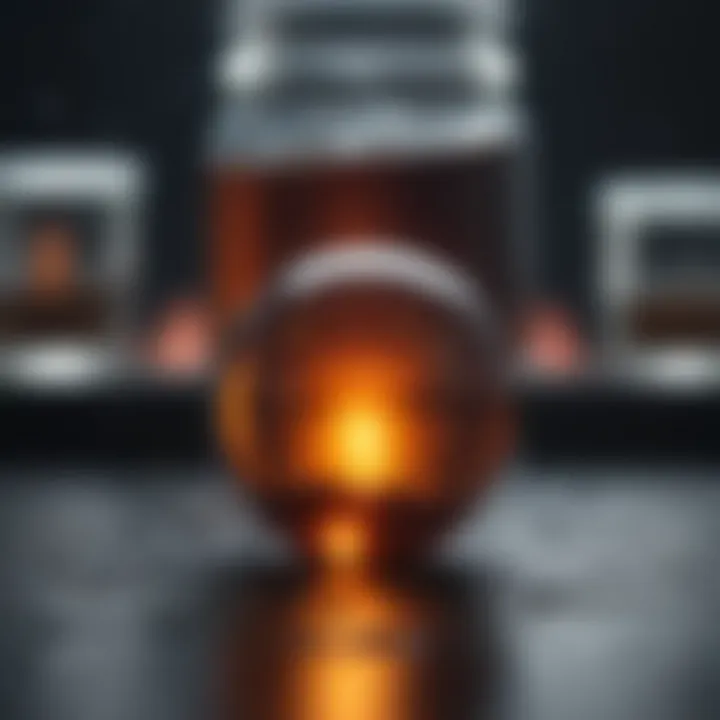
Materials Science Innovations
Types of Advanced Materials
In materials science, advanced materials such as composites, membranes, and nanomaterials lead the charge in technological innovations. Their unique properties vary from lightweight strength to adaptive responses to environmental changes, making them essential in today's competitive market.
A fundamental characteristic that distinguishes advanced materials is their multifunctionality—some materials can perform several functions, which increases efficiency and reduces manufacturing costs. This is particularly desirable in sectors like aerospace and automotive, where weight reduction is crucial for performance.
However, the complexity of producing these advanced materials can be a stumbling block, potentially hindering broader adoption across industries.
Chemical Processes in Material Design
The chemical processes involved in material design encompass a wide range of techniques from molecular synthesis to polymerization. These processes directly contribute to developing materials with desired properties tailored for specific applications.
One important characteristic is the scalability of these processes. Innovations in chemical manufacturing allow for smaller, more efficient operations that can still meet large-scale demands. This is especially significant in contexts where rapid production is key to keeping pace with consumer expectations.
A noteworthy benefit of advanced chemical processes is their potential for creating more sustainable materials through bio-based resources. However, one drawback lies in the stability and longevity of these new materials, challenging their long-term effectiveness in practical applications.
In sum, the applications of chemical products stretch from ensuring effective healthcare solutions to advancing agricultural practices and innovating materials science. Understanding each element's unique characteristics and challenges provides insight into the broader dialogue surrounding chemistry's role in shaping a sustainable future.
Case Studies in Chemical Products
Case studies serve as illuminating examples within the realm of chemical products. They showcase the practical applications of theoretical knowledge and emphasize the transformative impact chemistry has on various domains. Furthermore, analyzing real-world scenarios provides insight into the complexities and intricacies involved in product development and implementation. In this section, we delve into two key areas: successful drug formulations and innovations in green chemistry.
Successful Drug Formulations
Analysis of Notable Pharmaceuticals
The analysis of notable pharmaceuticals provides a window into how particular drugs have significantly changed healthcare landscapes. Take for instance the development of penicillin, recognized as one of the first true antibiotics. The structure of beta-lactam made it a game-changer in treating bacterial infections, saving countless lives. The key characteristic of this analysis is its historical context—understanding how past innovations set the stage for today’s drug formulations.
The examination of such pharmaceuticals is a beneficial choice for this article because it underscores the evolution of medicinal chemistry. Each drug comes with its own unique challenges and breakthroughs, illustrating the necessity for ongoing research and adaptability in the field. For example, while penicillin is widely celebrated, its overuse has led to resistance among bacteria, presenting a unique feature of the pharmaceutical world, where benefits can sometimes lead to unexpected downsides.
Chemistry Behind Drug Efficacy
The chemistry behind drug efficacy focuses on the molecular mechanisms enabling a drug to create its intended effect. For instance, the structure-activity relationship (SAR) is crucial in determining how well a drug interacts with its target. A notable example is the use of statins to lower cholesterol; these compounds inhibit HMG-CoA reductase, a key enzyme in cholesterol synthesis.
The key characteristic here is the intimate link between chemical structure and biological activity. This relationship not only informs drug design but also highlights why certain drugs are more effective than others. By understanding mechanisms at a molecular level, researchers can develop enhanced versions or entirely new drugs—this adaptability illustrates the benefits of thorough chemical understanding in drug formulation. However, the flip side is that this process often requires extensive testing and can lead to unexpected side effects.
Innovations in Green Chemistry
Case Studies Exemplifying Green Approaches
Examining case studies that exemplify green chemistry highlights innovative methods aimed at reducing waste and increasing sustainability. A compelling instance is the use of biocatalysis in producing pharmaceuticals, like converting vegetable oils into biodiesel. These cases often showcase enzyme-based reactions that occur with high specificity and fewer by-products, marking a significant shift toward more eco-friendly practices.
The key characteristic here is the focus on sustainability without sacrificing efficiency. For this article, it's a good choice to highlight these examples because they provide tangible proof that the industry can innovate while being environmentally responsible. The unique feature of biocatalysis is its lower energy requirement and milder reaction conditions compared to traditional methods, which can lead to significant cost savings over time. This, however, can be counterbalanced by challenges like enzyme stability that sometimes limit their application.
Impact on Environmental Sustainability
The impact of green chemistry on environmental sustainability is profoundly significant. Utilizing solvent-free processes and recycling materials are just two ways how innovations have reduced chemical footprints. For instance, the production of polylactic acid (PLA) from renewable resources like corn starch signifies not just advancement in material science but a conscious effort towards reducing reliance on fossil fuels.
The key characteristic of this impact lies in its dual focus on product functionality and ecological responsibility. Essentially, the trend is beneficial for the environment and meets the growing demand for sustainable practices. The unique feature of such sustainable approaches is their potential for integration with existing industrial frameworks, paving the way for broader acceptance. Nevertheless, the initial investment and technological hurdles can often complicate the transition.
The embrace of green chemistry underscores a pivotal shift in how chemical products are designed, leading to both economic and environmental advantages.
By analyzing these case studies, it’s evident that strategic choices in chemical synthesis and product formulation can lead to groundbreaking outcomes. The intersection of chemistry, innovation, and environmental responsibility motivates ongoing advancements in both drug development and sustainable practices.
Future Directions in Chemical Products
The progression of chemical products continues to evolve, driven by advancements in technology and a greater awareness of ethical and environmental responsibilities. The exploration of future directions not only sheds light on innovative practices but also paves the way for sustainable and efficient methods that can benefit society at large. In this section, we will delve into emerging trends and the influence of artificial intelligence on chemical research, which are crucial elements for understanding the future landscape of chemical products.
Emerging Trends in Chemistry
Nanotechnology Applications
Nanotechnology is changing the game in various fields, including medicine, electronics, and materials science. The unique aspect of nanotechnology is its ability to manipulate matter at the atomic or molecular level, leading to products with enhanced properties. This is significant because it creates materials that are not only stronger and lighter but also more effective in their intended applications.
Some of the key characteristics of nanotechnology include:
- Increased surface area leading to improved interactions in reactions.
- Enhanced physical and chemical properties that differ from their bulk counterparts.
Why is nanotechnology considered a beneficial choice? The benefits are numerous. For instance, in pharmaceuticals, nanoparticles can deliver drugs directly to targeted sites in the body, minimizing side effects. However, it is not without challenges. Issues related to safety, toxicity, and environmental impact remain concerns that need addressing.
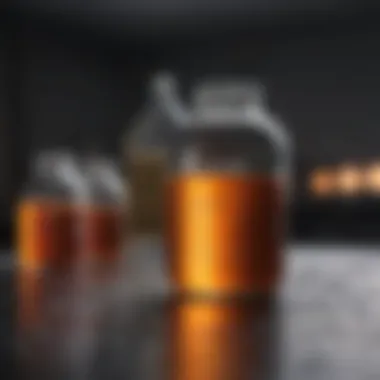
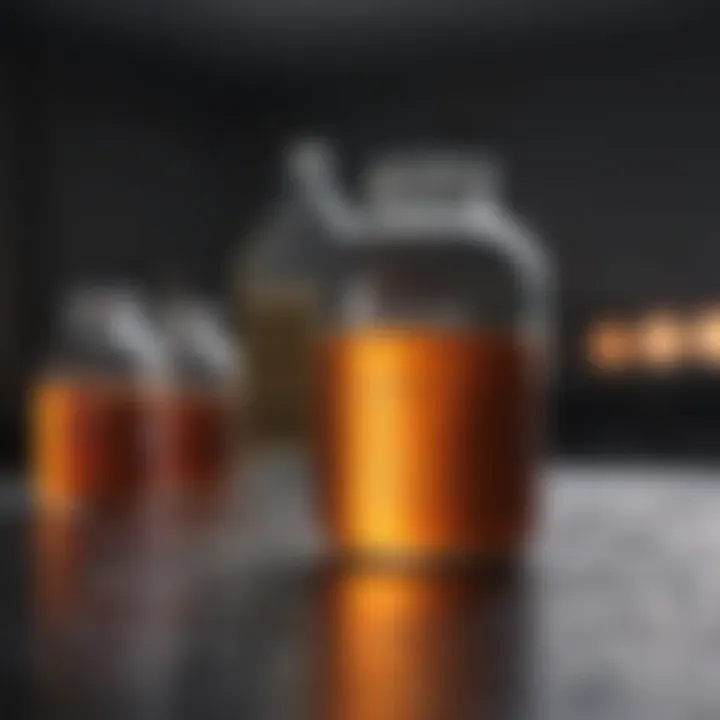
Continuous Flow Chemistry Developments
Continuous flow chemistry represents a significant shift from traditional batch processes. This method focuses on the steady flow of reactants through a reactor, allowing for more controlled reactions and increased efficiency. One standout feature is its scalability; processes can be easily adjusted to produce varying quantities, which is ideal for industries needing flexible outputs.
Key highlights of continuous flow chemistry include:
- Consistent product quality due to better temperature and mixing control.
- Reduced waste and energy consumption compared to traditional methods.
Adopting continuous flow chemistry is seen as a beneficial development in the landscape of chemical products. However, initial setup costs for continuous flow systems can be high, which may deter smaller organizations from transitioning. Nevertheless, as technology advances, these systems become more accessible and cost-effective.
The Role of AI in Chemical Research
Artificial intelligence is swiftly making its mark across various sectors, including chemical research. In recent years, AI-driven methods have shown the potential to accelerate discovery processes and optimize synthetic routes, thus enhancing productivity in chemical industries.
AI-driven Discovery Processes
AI-driven discovery processes focus on the use of algorithms and machine learning to predict the outcomes of chemical reactions. This means researchers can identify viable candidates for new products much faster than traditional methods. A standout feature is the sheer volume of data AI can analyze, allowing it to uncover patterns that could elude human researchers.
This approach is beneficial because it enables:
- Faster screening of chemical compounds, reducing time-to-market for new products.
- Increased precision in predicting successful reactions, saving resources in trial-and-error experiments.
On the flip side, the reliance on AI may lead to challenges as well. Issues such as algorithmic bias and data quality must be closely monitored to ensure reliable results.
Predictive Models in Chemical Synthesis
Predictive modeling in chemical synthesis takes the AI approach one step further. It uses simulations to forecast the behavior of chemical reactions under various conditions. This is vital for optimizing existing synthesis routes and discovering new ones. A significant characteristic of predictive models is their capability to incorporate vast data sets and complex chemical interactions into meaningful insights.
The advantages of these models include:
- Improved efficiency in the design of new synthetic pathways.
- Less reliance on empirical testing, which can be time-consuming and costly.
However, predictive models also face challenges. For instance, they may not always accurately represent real-world conditions, which can lead to discrepancies between predicted and actual outcomes. Researchers must balance computational predictions with practical experiments to ensure reliability.
Ultimately, the future directions in chemical products lie in embracing innovative technologies and methodologies, harnessing their potential while navigating the challenges they present.
Ethics and Safety in Chemical Production
In today's rapidly advancing technological landscape, the ethics and safety of chemical production have gained paramount importance. With the intricate balance between innovation and responsibility, stakeholders in chemistry—from researchers to manufacturers—need to understand that the implications of their actions extend well beyond the laboratory or factory floor. The products and processes employed in chemical production not only impact human health but also the environment. This section sheds light on critical aspects of ethics and safety, focusing on regulatory frameworks and health safety considerations.
Regulatory Frameworks
Global Regulations Governing Chemical Products
Regulatory frameworks play a crucial role in overseeing the production and distribution of chemical products globally. Various international bodies, such as the United Nations Environment Programme (UNEP) and the Organisation for Economic Co-operation and Development (OECD), establish guidelines to ensure the safety of chemical substances. These regulations help manage risks associated with chemical use, driving manufacturers to prioritize safety and compliance.
One of the key characteristics of these global regulations is their comprehensive nature, which encompasses a wide array of chemicals and their potential impacts. This broad scope not only facilitates a safer marketplace by minimizing hazardous exposures but also fosters public trust in chemical products. However, these regulations can vary significantly between countries, which can lead to confusion and inconsistencies in compliance—an important drawback that manufacturers must navigate carefully.
A unique feature of these regulations is the emphasis on hazard classification and labeling. Clear labeling ensures that end-users are well-informed about the safe handling and potential risks associated with chemicals, making it beneficial for promoting a safety culture. Yet, the extensive documentation and standardization required can also be viewed as burdensome, especially for smaller companies looking to innovate quickly.
Compliance and Industry Standards
Compliance with industry standards complements global regulations by offering more specific guidelines tailored to particular sectors. Organizations like the American National Standards Institute (ANSI) and the International Organization for Standardization (ISO) work to set the gold standard for safety practices within the chemical industry.
The critical aspect of compliance is its focus on continuous improvement and regulatory harmony among manufacturers. By adhering to established guidelines, companies not only mitigate risks of non-compliance but also enhance their reputation and consumer confidence. It is a popular choice as it helps streamline operations and boosts efficiency, ultimately benefiting the bottom line.
However, a unique challenge of compliance is the potential for evolving standards. As science and technology progress, so do the standards—sometimes leaving companies playing catch-up. This may hinder innovation in some instances but can also catalyze positive changes in safety practices.
Health and Environmental Safety
Risk Assessment Protocols
Effective risk assessment protocols form the backbone of health and environmental safety in chemical production. These protocols evaluate potential exposures to hazardous substances and identify the required safety measures. Risk assessments extend from the initial production phases to end-use applications, ensuring a holistic approach to safety.
A defining characteristic of these assessment protocols is their systematic nature, which involves detailed planning, data collection, and risk analysis. This empirical approach is beneficial because it provides concrete evidence that informs safety decisions and regulatory measures.
However, creating comprehensive risk assessments requires significant resources and expertise, which can be a disadvantage, especially for smaller organizations. Balancing thoroughness with practicality can be tricky, leading to potential oversights if not managed adequately.
Sustainable Practices in Chemical Manufacturing
Sustainable practices in chemical manufacturing are essential to reducing environmental impacts while ensuring worker safety. Concepts like greener chemistry and waste minimization have emerged as vital elements in this domain. These practices encourage manufacturers to rethink processes and materials, focusing on sustainability without compromising product quality.
The key aspect of sustainable practices is their focus on reducing the ecological footprint of chemical production. By minimizing waste and utilizing renewable resources, companies not only comply with regulations but also contribute positively to the planet. This is increasingly becoming a popular choice, appealing to environmentally conscious consumers and investors alike.
Nevertheless, transitioning to sustainable practices involves challenges, including higher initial costs and the need for specialized expertise. While it presents an opportunity for innovation, companies must weigh economic feasibility against environmental benefits.
"Ethics and safety in chemical production is not just a regulatory obligation; it is a moral imperative that shapes the future of our planet and society."
In summary, ethics and safety are essential considerations in the realm of chemical production. By navigating the intricate landscape of regulatory frameworks, compliance, risk assessments, and sustainable practices, industry players can foster a safer, more responsible approach to chemical production. The interaction between these elements provides a foundation for an ethical framework that benefits human health and the environment.