The Carbon Life Cycle: A Comprehensive Examination
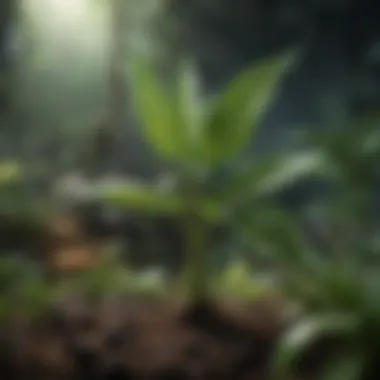
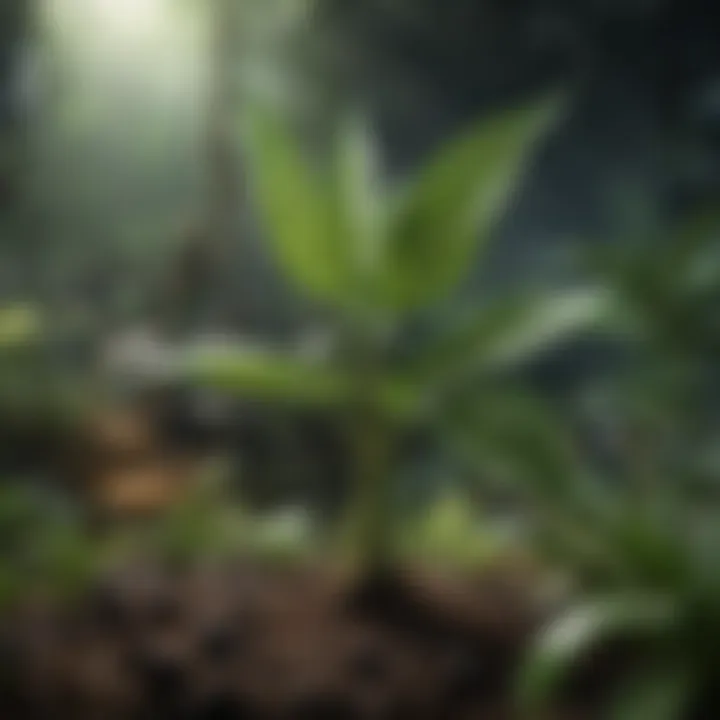
Intro
Understanding how carbon moves through our ecosystems is crucial in an age where climate change looms large over every conversation about environmental health. The carbon life cycle is intricate, involving various physical, chemical, and biological processes that let carbon exist in many forms and locations. Missing from this cycle, one may not only grasp the workings of our planet but also the profound implications for sustaining life.
Key Concepts and Terminology
This section breaks down the essential terms and concepts related to the carbon life cycle. Understanding these is the first step to grasping the nuances of how carbon influences both our atmosphere and biosphere.
Definition of Key Terms
- Carbon Fixation: This refers to the process by which inorganic carbon (usually in the form of carbon dioxide) is converted to organic compounds by living organisms, primarily through photosynthesis.
- Respiration: A process in which cells convert organic matter back into carbon dioxide, releasing energy for biological activities.
- Decomposition: The breakdown of dead organic material by various organisms, returning carbon to the atmosphere or soil.
- Carbon Sequestration: The long-term storage of carbon in a sink, such as forests or ocean sediments, preventing it from entering the atmosphere.
Concepts Explored in the Article
- The interplay between natural processes (like photosynthesis and respiration) and human actions (such as fossil fuel combustion and land use changes).
- The significance of carbon in different forms (e.g., CO2, methane, carbohydrates) and how these forms influence environmental quality.
- The role of carbon management strategies in mitigating climate change outcomes.
Findings and Discussion
As it turns out, understanding the carbon life cycle can unveil a myriad of insights that extend far beyond mere scientific curiosity.
Main Findings
Research has illuminated several pivotal aspects of the carbon life cycle:
- Interconnectedness: Every phase of the carbon cycle is linked; if one aspect falters, the entire system feels the effects. Deforestation, for example, not only releases stored carbon but also impacts local climates and biodiversity.
- Anthropogenic Impact: Human activities have dramatically altered the natural flow of carbon. Industrialization and agricultural practices have placed additional burdens on existing systems, hastening the effects of climate change.
- Ecological Implications: The findings suggest that simplistic, single-focus solutions are inadequate. Real success will hinge on comprehensive strategies that consider the carbon cycle's complexity and the dynamics of local ecosystems.
Potential Areas for Future Research
Several critical areas now beg further investigation:
- Evaluating the effectiveness of carbon sequestration techniques across various ecosystems.
- In-depth studies into the feedback loops present in climate models concerning carbon dynamics and their accuracy in predicting future scenarios.
- Examining carbon flow variations across different geographic and ecological contexts.
"The carbon life cycle is not merely a scientific concept; it is an existential narrative, intertwining the survival of our planet with our choices and practices."
For further insights and discussions surround the carbon life cycle, resources like Wikipedia and Britannica can be invaluable.
Intro to the Carbon Life Cycle
Understanding the carbon life cycle is crucial in today’s age, marked by increasing environmental awareness and climate considerations. The carbon life cycle involves the transition of carbon atoms through various forms and processes across different spheres in our ecosystem. This cycle plays a significant role in determining both the health of our planet and the atmospheric balance. By dissecting each component of the carbon life cycle, we can better comprehend the interplay between biological, geological, and human factors.
In essence, the examination of the carbon life cycle provides insights not only into environmental science but also the larger framework of planetary health.
Definition and Importance
The carbon life cycle refers to the continuous movement of carbon in various forms, like carbon dioxide and organic compounds, from the atmosphere to the Earth's crust and back again. This intricate journey encompasses key processes such as photosynthesis, decomposition, and the combustion of fossil fuels. The importance of understanding this cycle cannot be overstated.
Here are several reasons emphasizing its significance:
- Ecosystem Insights: It allows researchers to understand how ecosystems function, as carbon is a fundamental building block of life.
- Climate Regulation: Carbon dynamics directly affect greenhouse gas levels, influencing climate change.
- Resource Management: Insight into the carbon cycle informs strategies for sustainable resource use, potentially guiding policies on forestry, agriculture, and energy.
By grasping the nuances of carbon cycling, we can take steps toward mitigating detrimental environmental impacts while maintaining ecosystem vitality.
Historical Context
Historically, the concept of the carbon cycle can be traced back to early scientific thought. Initial recognition of carbon's crucial role in organic life dates as far back as the 18th century. Notably, Joseph Priestley and Antoine Lavoisier laid foundational work on gas exchanges involved in respiration and combustion processes.
The late 20th century marked a turning point as concerns regarding carbon emissions began to intertwine with broader discussions about environmental sustainability. Awareness around human-induced factors—such as fossil fuel consumption and deforestation—came to the fore, highlighting a pressing need to manage carbon emissions actively.
Today, the carbon life cycle is not just an academic topic but a public concern, with efforts being made globally to understand and reverse trends that contribute to climate change. As we dig deeper into each phase of the carbon cycle, the relevance of historical understanding becomes apparent, shaping contemporary debates on carbon management and environmental policy.
Carbon Sources
Understanding carbon sources is crucial as they form the foundation of the carbon life cycle. This section looks at how different sources contribute to the overall balance of carbon in the environment. Recognizing these sources helps in addressing climate change and managing carbon levels.
Natural Sources
Natural processes contribute significantly to the carbon balance on Earth. Among these, volcanic eruptions, ocean release, and respiration by living organisms play key roles.
Volcanic Eruptions
Volcanic eruptions introduce carbon into the atmosphere primarily through the release of carbon dioxide during explosive activities. This natural phenomenon can be powerful; a large eruption might release more CO2 in a few days than humans emit in a year. One key characteristic of volcanic eruptions is their ability to inject carbon along with ash and other gases at high altitudes, affecting atmospheric conditions over a vast area.
Unique Feature: The unpredictability of volcanic eruptions makes their carbon output variable, depending on the eruption's size and frequency.
Advantages/Disadvantages: While it’s a natural process, volcanic emissions contribute to short-term shifts in atmospheric composition, which can affect global temperatures. However, the overall contribution of these eruptions to climate change is relatively small in comparison to human activities.
Ocean Release
The oceans are an enormous reservoir of carbon, and they release it back into the atmosphere through processes like outgassing, where dissolved carbon dioxide escapes from ocean water. This mechanism is particularly significant when ocean temperatures rise. A notable aspect of ocean release is that it acts as a buffer for atmospheric carbon levels.
Unique Feature: The sheer capacity of oceans to store carbon is unmatched, and they cycle vast amounts of carbon daily.
Advantages/Disadvantages: While this process can keep atmospheric CO2 levels relatively stable, increased ocean temperatures due to climate change could lead to higher rates of carbon release, potentially exacerbating global warming.
Respiration by Living Organisms
All aerobic organisms, from tiny microbes to large mammals, respire and release carbon dioxide. Through this process, organisms break down carbohydrates and other compounds, releasing energy and CO2 as byproducts. This cycle is fundamental for maintaining life and promoting organic development.
Unique Feature: The concept of carbon cycling through respiration highlights the interconnectedness of life; when one organism respites, it plays a part in the grander scheme of ecosystem functions.
Advantages/Disadvantages: While respiration is essential for energy flow within ecosystems, it also contributes to the overall carbon cycle. The balance can be disrupted, however, when additional anthropogenic sources increase CO2 levels significantly.
Anthropogenic Sources
Contrarily, the carbon released by human activities underscores a growing concern for environmental sustainability. Three prominent human sources of carbon emissions include fossil fuel combustion, deforestation, and cement production.
Fossil Fuel Combustion
Fossil fuel combustion is a leading contributor to anthropogenic carbon emissions. By burning coal, oil, and gas for energy, we release large amounts of CO2 and other greenhouse gases into the atmosphere. This characteristic makes fossil fuel combustion a primary focus in discussions around mitigating climate change.
Unique Feature: The sheer volume of carbon emitted by fossil fuel usage has significantly outpaced any natural sources.
Advantages/Disadvantages: The advantage of fossil fuels lies in their ability to provide substantial energy for modern developments. However, the downside is their adverse effect on atmospheric carbon, leading to global warming and other negative environmental impacts.
Deforestation
Deforestation not only adds CO2 to the atmosphere by burning trees but also eliminates vital carbon sinks that previously absorbed this greenhouse gas. The loss of forests means a reduction in the planet's capacity to sequester carbon. This process can alter local climates and contribute to changes in weather patterns.
Unique Feature: Forests store carbon in their biomass and soil, thus their removal can have a lasting impact.
Advantages/Disadvantages: While deforestation may clear land for agriculture and development, it leads to increased carbon levels and biodiversity loss. This aspect makes it a critical issue in discussions surrounding carbon management.
Cement Production
Cement production is a significant industrial source of carbon emissions, contributing roughly 8% of the global total. The process of transforming limestone into lime releases CO2 as a byproduct. This industrial activity highlights a unique intersection of construction and environmental impact.
Unique Feature: The demand for cement is continuously growing, further compounding its environmental footprint.
Advantages/Disadvantages: Cement is essential for infrastructure development; however, its production emits carbon both through the chemical process and the energy consumed during manufacturing. Mitigating its impact necessitates innovations in material science and energy usage.
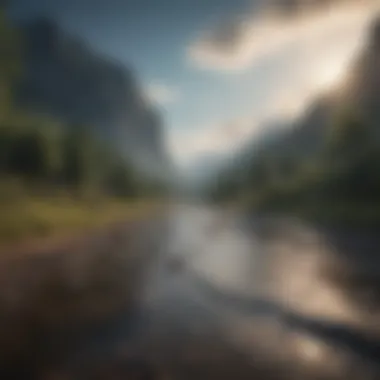
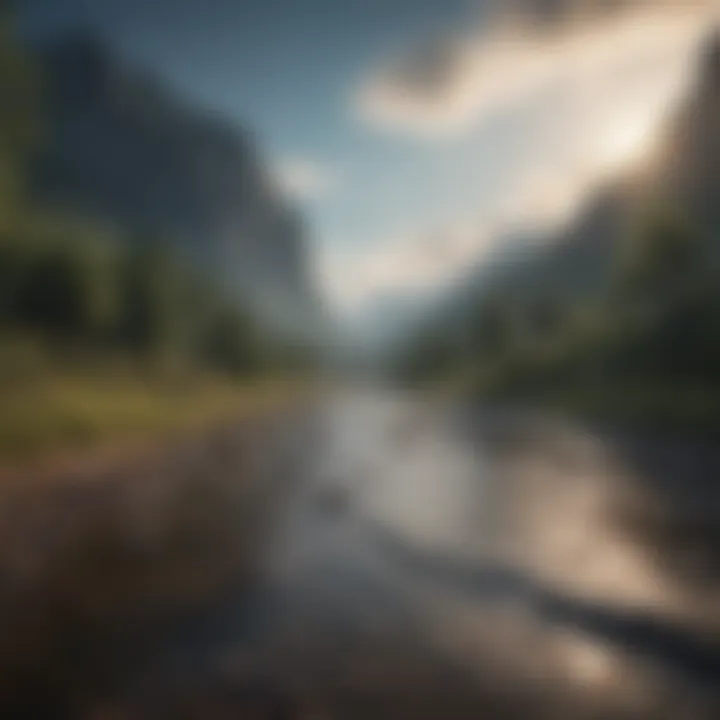
In summary, both natural and anthropogenic sources are integral to understanding the carbon cycle. Natural sources maintain a balance, while human activities largely disrupt it. Successfully addressing the challenges posed by these anthropogenic sources will be critical for future carbon management efforts.
Carbon Fixation
Carbon fixation is a crucial component of the carbon life cycle, serving as the primary pathway through which atmospheric carbon dioxide is converted into organic compounds. This process is foundational not just for plant growth, but also for sustaining the intricate web of life on Earth. By transforming carbon from its inorganic state into forms that can be used by living organisms, carbon fixation forms the basis of biological energy and food sources, influencing ecological dynamics and overall biome health.
The importance of carbon fixation extends beyond individual plants; its efficiency and capacity directly impact carbon levels in the atmosphere. Given the rising concerns over climate change, understanding carbon fixation helps inform strategies aimed at reducing atmospheric carbon levels. The interplay between photosynthesis and various carbon fixation pathways provides insights into how ecosystems can potentially thrive amid changing environmental conditions.
Photosynthesis Process
Role of Chloroplasts
Chloroplasts are essentially the powerhouses of photosynthesis. They house the machinery necessary for capturing light energy and using it to convert carbon dioxide and water into glucose and oxygen. These organelles contain chlorophyll, the pigment responsible for absorbing sunlight; this characteristic makes chloroplasts a pivotal aspect of green plants.
The chloroplast’s ability to harness sunlight is notable. It allows plants to produce their own food, making them autotrophs, which contributes significantly to the energy flow within ecosystems. The effectiveness of chloroplasts in facilitating photosynthesis highlights their essential role in not only supporting plant life but also in maintaining balanced atmospheric carbon levels. With climate dynamics in consideration, fostering healthy chloroplast functions can be a beneficial route for enhancing carbon fixation.
Carbon Dioxide Conversion
Carbon dioxide conversion is integral to the photosynthesis process. Here, the molecule is fixed into a more stable form of sugar through a series of biochemical reactions that occur in the chloroplasts. What stands out is the efficiency with which plants can absorb and utilize CO2, transforming it into organic material that serves as food.
This conversion process is particularly beneficial in that it supports life at multiple trophic levels. When plants fix carbon, they not only sustain themselves but also provide energy for herbivores, which, in turn, supports carnivores. In the context of the escalating climate crisis, appreciating the nuances of carbon dioxide conversion provides clear advantages. Enhancing this aspect of photosynthesis can lead to robust strategies that mitigate atmospheric carbon levels.
Alternative Carbon Fixation Pathways
While traditional photosynthetic pathways are vital, there are alternative methods like the C4 and CAM pathways that some plants utilize, especially in specific environments. These adaptations allow for more efficient carbon fixation under certain conditions, providing resilient strategies for thriving in diverse habitats.
C4 Pathway
The C4 pathway uniquely allows certain plants, like sugarcane and corn, to effectively concentrate carbon dioxide in their tissues. This method enhances photosynthetic efficiency, particularly in hot and arid climates where water loss is a concern. The key characteristic of the C4 pathway is its capacity to minimize photorespiration—an energy-wasting process that many plants face under stress.
By employing this pathway, producers can achieve higher yields compared to those using the traditional C3 photosynthesis. In light of growing global food demands and the necessity for climate adaptability, promoting the C4 pathway showcases great potential as a beneficial approach toward sustainable agriculture.
CAM Pathway
Similar to the C4 pathway but distinct in its functioning, the CAM pathway is utilized by plants like cacti and succulents in arid environments. This pathway allows for carbon fixation at night, when the temperatures are cooler, thus reducing water loss during the hot daytime. The CAM pathway’s key characteristic is timeliness; it emphasizes effective resource utilization by aligning gas exchange with environmental conditions.
While its unique adaptations provide significant advantages for survival in harsh climates, the CAM pathway also limits growth rates compared to C3 and C4 plants due to its slower processing of carbon. Understanding these dynamics presents both challenges and opportunities that can be leveraged in future research, potentially expanding our toolkit for carbon management amid varying environmental contexts.
"Understanding the processes of carbon fixation is essential for developing innovative strategies that can help counteract climate change and biodiversity loss."
In summary, comprehending the various facets of carbon fixation, from the roles of chloroplasts to the distinct pathways of C4 and CAM plants, is key to appreciating its significance in the carbon life cycle. The dialogue surrounding carbon management and climate resilience rests significantly on these foundations.
Carbon Storage
Carbon storage is a crucial component in the carbon life cycle, facilitating the capture and retention of carbon in various forms across different environments. This phase is particularly significant as it directly impacts greenhouse gas concentrations in the atmosphere. Through efficient carbon storage, ecosystems and human interventions can mitigate climate change and support biodiversity.
Terrestrial Carbon Stocks
Forests
When talking about forests, it's hard to overlook their immense role in carbon storage. They are often referred to as the lungs of the Earth due to their ability to absorb carbon dioxide during photosynthesis. A key characteristic of forests is their biomass; trees and vegetation act as significant carbon sinks. This feature makes them a popular subject of environmental studies, as protecting existing forests and restoring deforested areas can enhance their carbon storage capacity. Moreover, forests also provide numerous ecosystem services, such as habitat for wildlife, regulation of water cycle, and maintenance of soil health. However, one drawback is that they are susceptible to wildfires and deforestation, which can release stored carbon back into the atmosphere, negating their benefits.
Grasslands
Grasslands, though often overlooked, contribute significantly to carbon storage as well. These ecosystems store carbon in their deep root systems, which not only sequester carbon but also support soil health. A defining trait of grasslands is their adaptability to various climates. They typically have lower biomass than forests but can still store substantial amounts of carbon, making them an effective choice for carbon management strategies. The uniqueness of grasslands lies in their resilience to disturbance; they can recover quickly from events like droughts or grazing. However, land-use changes, such as agricultural expansion, can threaten these ecosystems, leading to a loss of stored carbon.
Soil Organic Matter
Soil organic matter is a vital aspect of carbon storage that often gets a bit of the limelight. It encompasses a mix of decomposed plant and animal material, which enriches soil health and biodiversity. One of its key characteristics is its ability to store carbon efficiently, with soils acting as one of the largest carbon reservoirs on land. This makes soil organic matter a beneficial focus for carbon management, especially considering its role in promoting fertile lands for agriculture. One unique feature here is that a healthy soil structure can retain water and nutrients better, ultimately supporting plant growth. Nevertheless, agricultural practices that disturb the soil excessively can lead to the degradation of organic matter, resulting in a potential increase in atmospheric carbon levels.
Oceanic Carbon Capture
Apart from terrestrial storage, the oceans also play an integral role in carbon capture. They not only absorb vast amounts of carbon dioxide but also participate in complex processes that regulate carbon dynamics.
Phytoplankton Role
Phytoplankton are microscopic organisms found in oceans and are fundamental in the carbon cycling process. They consume carbon dioxide during photosynthesis, contributing significantly to the ocean's capacity to absorb atmospheric carbon. A key characteristic of phytoplankton is their rapid growth and high turnover rate, which allows them to sequester carbon efficiently. This makes them a popular subject of marine biologists and environmentalists alike, as they are essential for understanding oceanic carbon dynamics. The unique aspect of phytoplankton is that they form the base of marine food webs, supporting a multitude of marine life. However, environmental stressors such as ocean acidification and rising temperatures pose threats to their populations, which could diminish their role in carbon storage.
Carbonate Sedimentation
Carbonate sedimentation represents another intriguing mechanism for oceanic carbon capture. This process involves the formation of calcium carbonate by marine organisms, such as corals and shellfish, which later contribute to long-term carbon storage in ocean sediments. A key characteristic of carbonate sedimentation is its ability to sequester carbon over geological time scales, essentially locking carbon away for millions of years. It's a beneficial strategy in the broader context of carbon management as it aids in stabilizing atmospheric carbon dioxide levels. The unique feature here is that carbonate sediments can also influence the chemical composition of seawater, with potential implications for marine ecosystems. Yet, increased ocean acidification can hinder the ability of these organisms to produce calcium carbonate, raising concerns about the sustainability of this carbon storage method.
Understanding these processes helps us to develop better strategies to mitigate climate change, protect ecosystems, and ensure a sustainable future.
Carbon Release Mechanisms
Understanding the mechanisms of carbon release is crucial to grasp the broader implications of the carbon life cycle. It encompasses the diverse processes through which carbon transitions from various forms back into the atmosphere. Not only does this shedding of carbon dictate the dynamics of climate change, but it also has significant consequences for ecosystem balance and health. Carbon release mechanisms serve as a natural part of carbon cycling; however, anthropogenic activities can amplify these processes, leading to environmental concerns.
Respiratory Processes
Respiration is a vital biological process that involves the conversion of glucose and oxygen into energy, releasing carbon dioxide as a byproduct. This occurs in nearly all living organisms, from tiny microbes to massive trees. The carbon released through respiration is a natural component of the carbon cycle, playing a role in regulating atmospheric CO2 levels. In ecosystems, the balance of photosynthesis and respiration is critical, as it dictates the overall carbon storage capacity.
In terms of ecosystem management, understanding the respiratory processes of different species can help in predicting the carbon emissions they may contribute, thereby aiding in conservation efforts and sustainability practices.
Decomposition
Decomposition is fundamentally the breakdown of dead organic matter, returning vital nutrients to the soil while releasing carbon dioxide into the atmosphere. It processes vital for nutrient cycling and soil health. Decomposition exhibits key mechanisms, which we'll dive into further.
Microbial Breakdown
Microbial breakdown is largely considered the unsung hero of decomposition. Microorganisms like bacteria and fungi break down complex organic materials, leading to the release of carbon dioxide. This process is highly efficient and vital for maintaining soil fertility. The key characteristic of microbial breakdown is its speed; it can rapidly transform organic matter into simpler compounds.
One unique feature is that microbial communities can adapt to different substrates, enhancing their efficiency across varying environments. Their effectiveness in nutrient recycling is advantageous; however, increased temperatures due to climate change can lead to faster decomposition, hence more carbon released than what ecosystems can handle – a potential tipping point for climate impacts.
Nutrient Cycling
Nutrient cycling involves the transfer of essential nutrients, such as nitrogen and phosphorus back into soil and living organisms, often aided by decomposition. The key characteristic of nutrient cycling is its ability to sustain plant and microbial life by maintaining nutrient availability. This is beneficial as it supports agricultural productivity as well as natural ecosystems.
A unique feature is the role of mycorrhizal fungi, which form symbiotic relationships with plant roots, improving nutrient uptake while also promoting soil carbon storage. The challenge arises when land-use changes disrupt these cycles, potentially leading to nutrient depletion and affecting ecosystem resilience.
Human-Induced Emissions
Human-induced emissions have escalated in recent decades due to industrialization and urbanization. These emissions alter the natural balance of the carbon cycle, adversely impacting our climate and ecosystems.
Combustion of Biomass
The combustion of biomass, while sometimes viewed as a carbon-neutral process, contributes a considerable amount of carbon dioxide and other emissions. Biomass burning has its roots in historical practices for energy, but it poses challenges today, particularly in terms of air quality and greenhouse gas concentrations. Its key characteristic is that it releases stored carbon quickly compared to the slower, natural processes of decay.
What makes it a point of discussion is the trade-off between energy production and air pollution. Biomass can provide renewable energy, but its impact on carbon levels needs consideration to avoid exacerbating climate issues.
Land Use Changes
Land use changes, such as deforestation or urban development, significantly influence carbon release. These alterations disrupt the carbon storage capabilities of ecosystems. The key characteristic of land use change is that it often results in immediate carbon emissions from the soil and vegetation, sometimes leading to long-term loss of carbon sinks.
For example, removing forests not only releases stored carbon but also diminishes the area's ability to sequester future carbon. Also, these changes can contribute to habitat destruction, affecting both biodiversity and ecosystem resilience. Understanding these processes is crucial for effective carbon management strategies.
Carbon Cycling in Ecosystems
The concept of carbon cycling in ecosystems is what keeps our planet alive. Carbon is not just a building block of life; it is a key player in ecosystem functions. By cycling through various biomes and communities, it ensures that energy flows and matter cycles are balanced. This intricate choreography benefits both species and habitats while influencing global processes such as climate regulation.
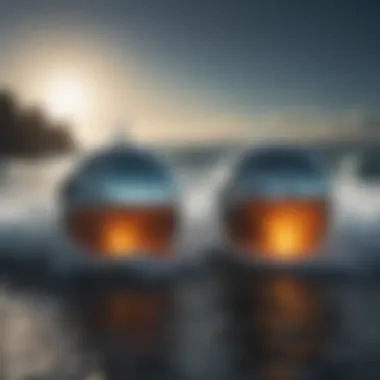
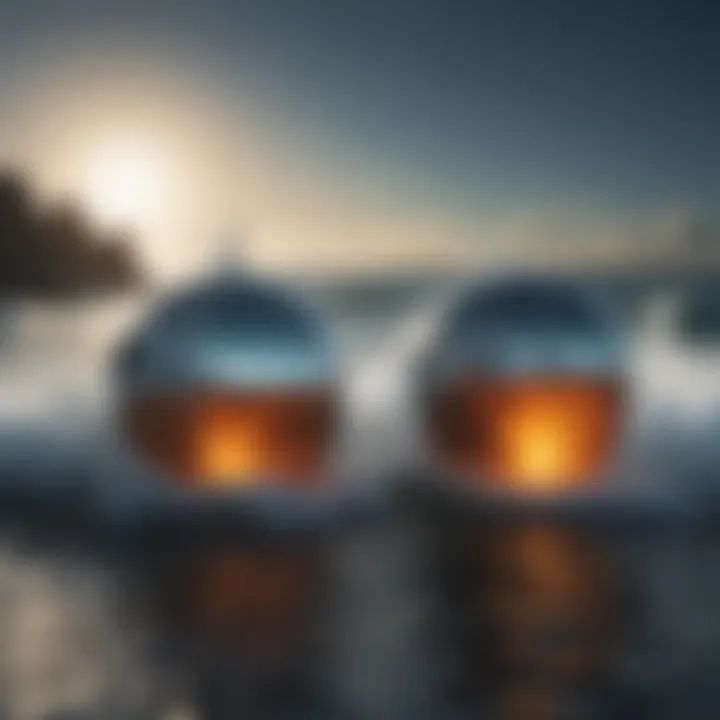
Role of Biomes
Understanding how different biomes interact with the carbon cycle helps to illuminate how ecosystems function. Each biome, with its unique characteristics, plays a vital role.
Tropical Rainforests
Tropical rainforests are often dubbed the "lungs of the Earth." This is not just a catchy phrase; it underscores their substantial contributions to carbon fixation. With their dense vegetation, these forests draw down large amounts of carbon dioxide from the atmosphere. The key characteristic of tropical rainforests is their year-round warm temperatures and high rainfall, which create ideal conditions for diverse plant life.
- Advantages:
- High biological diversity contributes to carbon retention.
- Complex root systems help in stabilizing soils, preventing erosion and facilitating nutrient cycling.
However, deforestation in these areas poses significant threats. When trees are removed, stored carbon is released back into the atmosphere, exacerbating climate change.
Deserts
Deserts might seem like ecological dead zones, but they have unique functions in the carbon cycle too. Their arid conditions may limit vegetation, but they still play a vital role in the global carbon budget. One notable aspect of deserts is their ability to sequester carbon in soil.
- Key Characteristic: The low organic matter in desert soils might surprise you. Yet, it can store carbon efficiently because the decomposition process is slower in dry conditions.
Though deserts contribute less to carbon storage compared to forests, their importance shouldn't be underestimated. They offer unique ecosystems that play a role in global carbon dynamics.
Tundra
The tundra is a surprising player in carbon cycling, often overlooked due to its harsh climate. Characterized by frozen subsoil, known as permafrost, this biome stores vast amounts of organic carbon. The key feature of tundra ecosystems is how they manage to retain carbon even in extreme cold. Even though the growing season is short, the capacity of tundra vegetation, primarily mosses and lichens, to capture carbon is quite significant.
- Advantages:
- The cold temperatures slow decomposition, conserving carbon in the soil.
- Increased plants during the brief summer help in capturing carbon before winter takes hold.
However, there’s an alarming issue with tundra ecosystems. As global temperatures rise, permafrost melts, releasing stored carbon into the atmosphere and potentially creating a feedback loop that accelerates climate change.
Impact of Biodiversity
Biodiversity enhances ecosystem functions, making it key to understanding carbon cycling. This healthy interplay among species leads to more robust systems.
Species Interactions
Species interactions in ecosystems are like a finely tuned machine. The relationships between different organisms, whether mutualistic, competitive, or even predatory, create the conditions that influence carbon flow.
- Key Characteristic: This dance of species ensures that resources such as light and nutrients are utilized more efficiently.
In the context of carbon cycling, one can’t overlook the benefit of diverse plant life that captures and stores more carbon as they produce biomass.
Ecosystem Resilience
Ecosystem resilience is about how well an ecosystem can bounce back from disturbances. High biodiversity boosts this resilience, securing the carbon cycle against shocks.
- Key Characteristic: Resilient ecosystems maintain functionality even under stress, ensuring that carbon storage and release are optimized.
Ensuring resilience not only helps in maintaining carbon levels but also protects against loss of biodiversity, which is critical for ecosystem health.
Understanding the roles of different biomes in the carbon cycle reveals how interconnected our world is. The delicate balance formed by ecosystems emphasizes why protection and restoration efforts are crucial.
Impact of Climate Change on Carbon Dynamics
The effects of climate change ripple through the intricate web of carbon dynamics, influencing everything from ecosystems to human society. As the planet warms, we face an evolving landscape where carbon compounds might behave in unexpected ways. Understanding how climate change alters the carbon life cycle is not just a theoretical exercise—it has real and urgent implications for our collective future.
Feedback Loops
Positive Feedback Mechanisms
Positive feedback mechanisms can significantly amplify the impacts of climate change, creating a chain reaction that escalates the effects on carbon dynamics. For example, as the Arctic ice melts due to rising temperatures, the earth’s surface becomes less reflective, absorbing more heat. This leads to further warming and more ice melting—a vicious cycle. This characteristic of positive feedback makes it extremely concerning in the context of global warming, as it may push our climate system into a state that is hard to reverse.
A unique feature of these mechanisms is their capacity to accelerate changes in ecosystems, which can have cascading effects on biodiversity. While some argue they could lead to adaptive changes in nature, the reality often leans toward disruptions and loss of species. Hence, the advantages of accelerating ecological processes are overshadowed by the potential for ecological collapse, positioning positive feedback as a complex challenge to address.
Negative Feedback Effects
On the flip side, negative feedback effects can help mitigate some of the more severe impacts of climate change. For instance, as CO2 levels rise, some plant species may thrive, increasing photosynthesis and drawing down carbon from the atmosphere. This aspect serves as a potential buffer in our climate system, providing a degree of resilience against the escalation of warming.
The key characteristic of negative feedback is its ability to stabilize systems. While not a cure-all, it presents beneficial opportunities for carbon management strategies. Retaining healthy forests and wetlands can foster these stabilizing responses. However, there are disadvantages; reliance solely on negative feedback mechanisms without addressing the root causes of emissions might lead to complacency, preventing necessary action against climate change.
Global Warming Consequences
Melting Permafrost
Melting permafrost presents one of the scariest aspects of climate change, constituting a major problem for carbon dynamics. As the ground thaws, it releases trapped carbon and methane, potent greenhouse gases that can amplify warming. When ice melts away, what was once a frozen carbon stock can become a source of emissions, turning stored carbon back into a contributor to climate change.
One key feature of this phenomenon is the potential for long-term implications. The sheer volume of organic matter contained in permafrost means that the consequences could span decades and beyond, impacting global warming on a scale that is hard to predict. The main disadvantage here is that it can create a relentless feedback loop, making it difficult to reins in future impacts.
Ocean Acidification
Ocean acidification is another alarming consequence of climate change. As CO2 levels rise in the atmosphere, much of it is absorbed by our oceans. This absorption alters the chemistry of seawater, impacting marine life, especially organisms that rely on calcium carbonate, such as corals and shellfish. This characteristic of ocean acidification points to its broad-reaching effects within marine ecosystems, affecting food chains and biodiversity.
One notable feature is that the repercussions of ocean acidification extend beyond the oceans themselves. They can influence global fish stocks, affecting food security for millions of people. While ocean ecosystems are remarkably resilient, the added stress from climate change poses substantial risks. Failure to mitigate these effects could unravel decades of marine and fisheries management, posing a formidable challenge for future generations.
"The melting of permafrost and the acidification of oceans are echoes of climate change that we must address collectively, lest we lose our footing in an uncertain future."
Strategies for Carbon Management
The topic of carbon management is pivotal in the context of climate change and environmental sustainability. It encompasses various methods and practices aimed at reducing carbon emissions and enhancing carbon sequestration. With growing awareness of the threats posed by climate change, strategic management of carbon has become more relevant than ever. This article provides insights into methods to tackle the carbon footprint effectively, ensuring a healthier planet for future generations.
Reduction in Emissions
Renewable Energy Adoption
The push for renewable energy adoption plays a significant role in reducing carbon emissions. By shifting away from fossil fuels and utilizing resources such as solar, wind, and hydroelectric power, we can significantly lessen the amount of carbon dioxide (CO2) released into the atmosphere. One of the remarkable aspects of renewable energy is its sustainability; it replenishes naturally and continuously.
Adopting renewable energy solutions not only reduces dependency on finite resources but also tailors the energy portfolio towards greener alternatives. Additionally, this transition can spark job creation in emerging sectors, enhancing local and national economies.
However, there are challenges in implementation. Initial investments can be steep, and the intermittency of certain renewable sources, like solar or wind, can create reliability issues. Thus, while the long-term benefits greatly outweigh these disadvantages, careful planning is essential.
Carbon Pricing Mechanisms
Carbon pricing mechanisms are indispensable for incentivizing reductions in emissions. They include strategies like carbon taxes or cap-and-trade systems. How they work is simpler than one might think; these mechanisms assign a cost to carbon emissions, encouraging businesses and individuals to reduce their carbon footprints. The beauty of carbon pricing lies in its flexibility—companies can choose their approach to cut emissions based on their unique circumstances.
Adopting these mechanisms has gained popularity because they align economic goals with environmental responsibility. However, there’s a unique challenge here: if set too low, they may not effectively encourage significant reductions. Strong political will and public support are generally necessary to establish and maintain effective pricing mechanisms.
Enhancing Carbon Sequestration
Reforestation and Afforestation
Reforestation and afforestation are powerful strategies for enhancing carbon sequestration. Reforestation involves restoring trees in deforested areas, while afforestation refers to planting trees in areas that have never been forested. Trees act as natural carbon sinks, absorbing CO2 from the atmosphere and storing it in their biomass and the soil beneath. One crucial characteristic of this approach is biodiversity; healthy forests provide habitat for countless species, enhancing ecosystem stability.
The long-term benefits of reforestation and afforestation are profound; these initiatives not only sequester carbon but also help combat soil erosion, improve water quality, and foster resilience against climate change. Nevertheless, there’s a flip side: the process can take decades to yield significant benefits, and careful management is needed to avoid introducing non-native species that could disrupt local ecosystems.
Soil Management Practices
Soil management practices significantly contribute to carbon sequestration as well. Practices like no-till farming, cover cropping, and organic matter addition can increase the organic carbon content of soils. The beauty of this method is its dual benefit: while it captures carbon, it also improves soil health, which can elevate agricultural productivity.
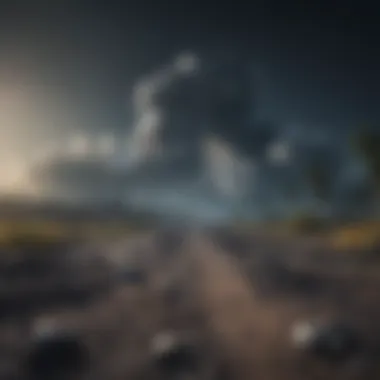
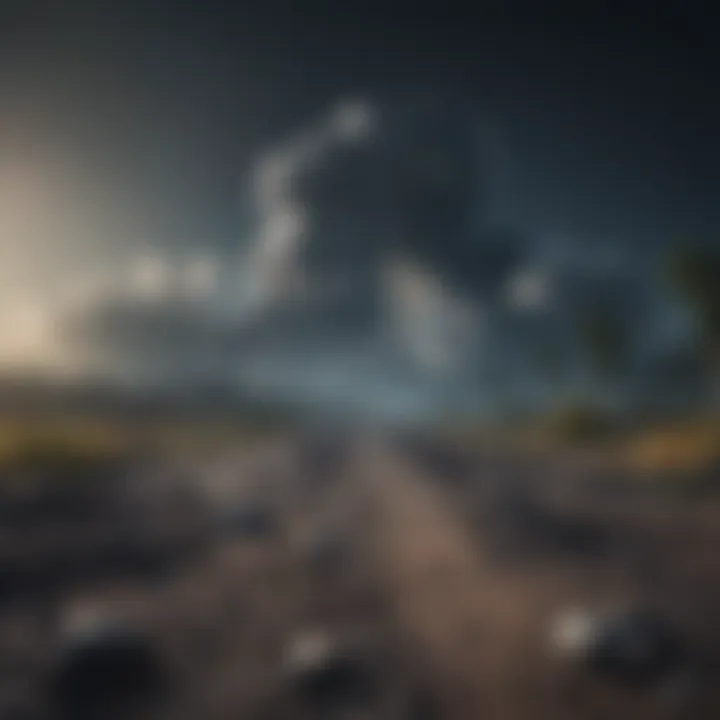
Soil's unique feature lies in its capacity to store vast amounts of carbon—more than the atmosphere and forests combined. However, sustainability is the name of the game; practices must be maintained over time. An additional challenge is that changing agricultural practices can face resistance from established farming communities who are accustomed to conventional methods.
"Effective carbon management strategies can bridge the gap between environmental protection and economic growth, fostering a more sustainable future."
In summary, the roadmap for effective carbon management passes through reducing emissions and enhancing sequestration. Different strategies, each with advantages and disadvantages, must be carefully evaluated and tailored to fit specific regional and local contexts. The intersection of environmental practices, policy, and human behavior remains a focal point in understanding and addressing global carbon dynamics.
Technological Innovations in Carbon Management
Technological innovations play a pivotal role in addressing the pressing challenges of carbon management. As awareness of carbon’s impact on climate change has grown, so has the need for cutting-edge solutions to curtail its detrimental effects. These technologies not only aid in reducing emissions but also enhance our ability to sequester carbon effectively.
Advancements in this field have led to various methods that combine scientific knowledge with practical applications, potentially reshaping our approach to environmental management. Let’s dive deeper into two prominent innovations: Carbon Capture and Storage (CCS) and Enhanced Weathering Techniques.
Carbon Capture and Storage (CCS)
Process Overview
Carbon Capture and Storage (CCS) is often seen as a beacon of hope in our collective aim to mitigate climate change. The process involves capturing carbon dioxide (CO2) emissions at their source—typically from power plants or industrial sites—before it can reach the atmosphere. Once captured, this CO2 is transported, usually via pipelines, and stored underground in geological formations, effectively locking it away from the environment.
One of the key characteristics of CCS is its scalability. Existing infrastructure can often be retrofitted to include carbon capture systems, which makes this a beneficial choice for many industries seeking to reduce their carbon footprint without overhauling operations completely. The unique feature of CCS is its capacity to handle large volumes of CO2, which is crucial given the vast emissions produced by fossil fuel-based energy production. However, the approach does come with disadvantages like high operational costs and the potential risks associated with long-term underground storage, which still require thorough evaluation.
Challenges and Future Prospects
Despite its potential, CCS faces a range of challenges. For starters, public perception can be a significant hurdle. Many communities are understandably concerned about the risks associated with geological storage, which has led to suspicions and delays in implementing CCS projects. Additionally, the technology is currently expensive to install and operate, which raises questions about its economic feasibility.
Yet, the unique feature of the challenges surrounding CCS is the rapid technological advancements being made. Innovations are emerging to lower costs and increase efficiency, making it a more attractive option for businesses. The future prospects for CCS are optimistic, especially with growing commitments from governments and industries alike to adopt green technologies. Ultimately, if these challenges are addressed head-on, CCS could become a crucial component in global carbon management efforts.
Enhanced Weathering Techniques
Enhanced weathering refers to a geoengineering strategy that aims to accelerate the natural processes through which minerals react with CO2 and draw it out of the atmosphere. This method involves spreading finely crushed silicate rocks, such as basalt, over land or ocean surfaces, where they react with CO2 to form stable carbonates.
The core of enhanced weathering lies in its dual benefit: it not only sequesters carbon but also enriches soils and enhances biodiversity. In agricultural settings, these techniques can improve yield while contributing to the global fight against climate change. However, the unique challenge here is the scalability of such methods, as they require substantial amounts of rock material and energy, which can pose logistical challenges.
The potential for enhanced weathering is indeed vast, but further research is needed to optimize its efficiency and assess its ecological impacts comprehensively. As with any technological innovation, a rigorous evaluation of long-term effects and viability in various environments is essential.
By embracing these technological innovations, we position ourselves to make significant strides in carbon management, helping to balance the carbon life cycle more effectively.
"Innovative approaches in carbon management are not just about reducing emissions but also about reshaping our future through sustainable practices."
The Role of Policy and Governance
The governance of carbon management plays a vital role in shaping the pathways through which carbon is managed across various levels. Policies influence how carbon emissions are regulated, how resources are allocated, and how nations engage in collaborative efforts to mitigate climate change. Effective governance provides a framework for dealing with carbon emissions, supporting sustainable development, and encouraging innovation in carbon capture and storage technologies.
International Agreements
Paris Accord
One of the key international frameworks in the fight against climate change is the Paris Accord. This agreement dovetails the efforts of almost every country in the world toward a common purpose: to keep global warming below two degrees Celsius above pre-industrial levels. The most significant aspect of the Paris Accord is its principle of nationally determined contributions (NDCs), allowing countries to tailor their commitments to their own circumstances while holding them accountable to a global standard.
The Accord is particularly beneficial for this article as it accentuates collaboration among nations. A unique feature of the Paris Accord is that it relies on transparent reporting mechanisms, which create a level of trust and ensure accountability among the signatories. However, some critics argue that without enforceable penalties, the Accord could falter—it remains to be seen whether it can successfully motivate compliance in the long term.
United Nations Framework Convention
The United Nations Framework Convention on Climate Change (UNFCCC) is another cornerstone of international climate action, aimed at addressing the various facets of climate change including carbon management. Its primary role is to facilitate negotiations among member states and provide a platform for climate governance. A notable aspect of the UNFCCC is its ability to adapt to new scientific findings and changed socio-economic conditions, which keeps its strategies relevant.
The UNFCCC stands out for its inclusiveness and dedication to participation across diverse economies, allowing nations of different sizes and development levels to contribute meaningfully to global climate strategies. This characteristic makes it a desirable choice in this narrative, as it promotes global engagement. However, the challenge remains in harmonizing these diverse efforts to achieve a unified and effective response to climate change—in some cases, this can lead to slower implementation of carbon management measures.
National Strategies
Emission Reduction Targets
National Strategies are critical in setting the groundwork for effective carbon management. In particular, emission reduction targets serve as explicit markers for nations to aim at. These targets can drive innovation in clean energy technologies and encourage collective societal shifts towards lower carbon footprints. A key characteristic of emission reduction targets is their specificity—governments outline expected reductions, creating not just goals but defined timelines to achieve them.
These targets are often seen as beneficial because they enable accountability—communities can track their country's progress and advocate for continuous improvement. One downside, however, is that if targets are not grounded in scientific reality, they can lead to public disillusionment, particularly if the goals seem unattainable.
Legislation for Green Technologies
Legislation for green technologies plays a vital role in fostering an environment conducive to innovation. By providing incentives like tax breaks or grants, governments can stimulate investment in renewable energies and advanced carbon capture solutions. The unique feature of such legislation is its potential to reduce barriers for new technologies and help industries transition away from fossil fuels.
In this article, legislation concerning green technologies is favored for its transformative capacity—it can spark job creation and economic growth in emerging sectors. However, an aspect that warrants caution is the need for a careful balance; overly stringent regulations might stifle innovation or drive industries overseas where regulations are lax, compromising the goal of effective carbon management.
Future Directions in Carbon Life Cycle Research
As the planet grapples with escalating climate concerns, the future directions in carbon life cycle research are crucial. This topic shines a light on how ongoing studies and innovations shape our understanding and management of carbon dynamics. By identifying emerging areas of research and collaboration, this section highlights the potential to improve carbon management practices significantly.
Emerging Research Areas
Integration with Land-Use Planning
The integration with land-use planning stands out as a beneficial aspect in our pursuit of effective carbon management. This crossfield approach marries urban development with ecological preservation, ensuring that land is utilized in a manner that considers both carbon storage and release. Land-use planning offers a roadmap—strategically guiding development to minimize habitat loss while promoting reforestation and sustainable agriculture.
One key characteristic of integrating land use with carbon management is its emphasis on zoning regulations that favor green spaces. Parks and urban forests can act as carbon sinks, contributing positively to local and global carbon cycles.
However, this approach isn't without challenges. Balancing development and conservation can be contentious, often igniting disputes among stakeholders. Moreover, inadequate data for effective planning can lead to miscalculations, resulting in suboptimal outcomes. The unique feature here is that planning must be adaptable; a successful land-use strategy will need to evolve based on ongoing research and environmental changes, making its implementation a delicate operation.
Impact Assessment Models
Impact assessment models play a pivotal role in predicting the effects of various land-use scenarios and carbon management strategies. These models utilize complex algorithms and data-driven approaches to assess potential emissions, risks, and benefits linked to human activities.
The key characteristic of these assessments lies in their ability to simulate environmental changes. For instance, they can illustrate how different carbon sequestration methods might impact local ecosystems over time. This predictive capability makes them invaluable for policymakers and planners aiming to mitigate climate change.
On the flip side, the sophistication of impact assessment models can be a double-edged sword. They require considerable computational power and often rely on vast datasets, which may not always be readily available. Additionally, these models can sometimes oversimplify or overlook critical variables, leading to uncertainty in their predictions. Nonetheless, their integration into carbon management strategies can guide institutions towards more informed decisions.
Collaboration Across Disciplines
Interdisciplinary Research Initiatives
Interdisciplinary research initiatives mark a significant shift in addressing carbon dynamics. This collaborative approach brings together experts from diverse fields—ecology, economics, urban planning, and more—to foster comprehensive understanding and innovative solutions. By pooling knowledge, disciplines can cover aspects of carbon management that may be overlooked by a more singular focus.
One defining trait of these initiatives is their emphasis on shared goals, encouraging collaborative projects that address climate challenges holistically. This multifaceted lens is proving popular as it enables the integration of differing methodologies and perspectives in tackling the complex nature of carbon cycles and management.
However, managing interdisciplinary teams can entail challenges surrounding communication, varying terminologies, and conflicting methodologies. Ensuring that all parties work towards a common language and goal is essential, albeit sometimes difficult to achieve. Nevertheless, the potential rewards of enhanced innovation and understanding in carbon dynamics render this approach particularly valuable.
Global Research Networks
The establishment of global research networks represents another vital direction in carbon life cycle research. These networks connect researchers, institutions, and policymakers worldwide, facilitating the sharing of knowledge, data, and best practices. Such connectivity ensures that efforts in carbon management are informed by a diverse array of perspectives and experiences.
A key characteristic of these networks is their commitment to open-access data and collaborative projects. This element is instrumental—having one central hub for information allows for cross-pollination of ideas and methodologies, driving progress faster than isolated research efforts.
Nevertheless, global networks can pose challenges related to standardization. Differences in data collection methods or research focus might lead to inconsistencies. Additionally, varying degrees of resource availability can create inequities in participation, although creating structures to ensure inclusion can help mitigate this issue. Ultimately, global networks are crucial for paving the way forward in carbon management and fostering solidarity in the face of climate change.
End
In summarizing the complex narrative of carbon's journey through various stages and processes, it becomes evident that understanding the carbon life cycle is imperative for multiple reasons. At a fundamental level, delving into this topic not only elucidates the mechanisms through which carbon is fixed, stored, and released but also highlights its critical role in maintaining ecological balance and regulating our climate. Moreover, it fosters a greater appreciation for the delicate interplay between natural systems and human activities.
Key Takeaways
- Interconnectedness of Processes: The carbon life cycle illustrates how different processes—such as photosynthesis, decomposition, and human activities—are deeply interconnected. Each segment influences the next, forming a web of interactions that can significantly impact ecosystems and climate.
- Human Impact: Human activities, particularly since the Industrial Revolution, have disrupted the natural carbon cycle. Recognizing and mitigating this disruption is essential for sustaining our environment.
- Nature’s Engineering: Natural processes, like the roles of forests and oceans in carbon sequestration, showcase nature's remarkable ability to regulate carbon levels. Advocating for the protection of these systems is crucial for future resilience.
- Policy Implications: The insights gained from studying the carbon life cycle have significant implications for policy-making. Effective strategies can be devised to reduce carbon emissions, enhance sequestration efforts, and ultimately combat climate change.
The Importance of Continuous Study
The necessity for ongoing research in the dynamics of the carbon life cycle cannot be overstated. Given the rapid changes occurring in our climate and ecosystems, continuous study allows for:
- Adaptation to Changes: Understanding evolving threats, such as shifting carbon sinks and unpredictable ecological responses, equips us to respond proactively.
- Refinement of Strategies: An ongoing examination reveals areas for improvement in carbon management practices, ensuring that they remain relevant and effective against new challenges.
- Integration of Disciplines: Carbon dynamics are not isolated to environmental science. Insights from behavior psychology, economics, and sociology are also crucial, fostering a holistic approach to problem-solving.
In essence, the study of the carbon life cycle serves as a cornerstone for informed decision-making and effective policy development aimed at preserving both our planet and future generations.