In-Depth Exploration of 3D Organoid Culture Protocols
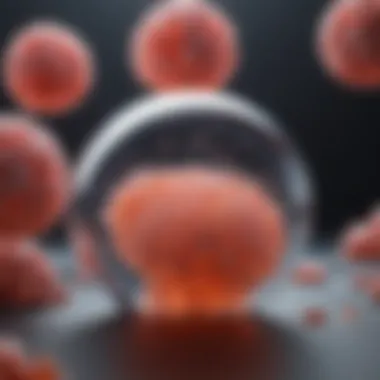
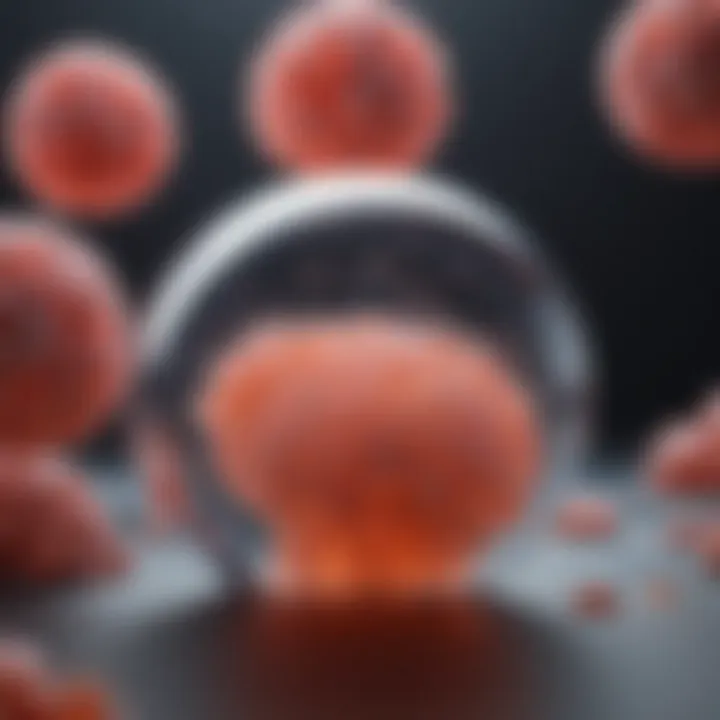
Intro
3D organoid culture protocols represent a rapidly growing field in biomedical research, offering innovative avenues for studying complex biological systems. These protocols enable the cultivation of miniature, organ-like structures that mimic the functionality and architecture of real tissues. This article aims to provide detailed insights into these protocols, their applications in research, and the challenges faced by scientists in this field.
Understanding organoids is essential for researchers and educators engaged in biomedical studies. The ability to create organ-like models allows for more accurate evaluations of disease mechanisms and drug responses than traditional 2D cell cultures.
In this piece, we will explore the foundational concepts and terminology associated with 3D organoid culture, discuss key findings, and highlight potential areas for future research. This comprehensive guide is tailored for students, researchers, and professionals seeking to deepen their knowledge in this vital area of study.
Key Concepts and Terminology
Definition of Key Terms
- Organoids: Miniaturized and simplified versions of organs, developed from stem cells or progenitor cells. They can self-organize into structures that replicate certain features of their in vivo counterparts.
- 3D culture: A method of growing cells in three dimensions, as opposed to on a flat surface, allowing for a more natural cell environment and better mimicry of organ function.
- Stem Cells: Undifferentiated cells that have the potential to develop into various cell types. They are often used as the basis for organoid development.
- Differentiation: The process by which stem cells become specialized cell types, essential for the formation of organoids.
Concepts Explored in the Article
The article covers several key concepts related to 3D organoid culture protocols:
- Protocol Steps: A systematic breakdown of the steps required to culture organoids, from cell preparation to maintenance and analysis.
- Types of Organoids: Insight into various organoids, including intestinal, liver, and brain organoids, highlighting their unique characteristics and uses.
- Applications: Examination of how these organoids are utilized in disease modeling, drug testing, and understanding developmental processes.
Findings and Discussion
Main Findings
3D organoid culture protocols offer researchers a powerful tool for investigating biological processes and disease states. Key findings from studies indicate:
- Organoids closely resemble the architecture and function of corresponding tissues, allowing for more accurate experimental results.
- They serve as effective models for studying disease mechanisms, including cancer and genetic disorders, providing insights into therapeutic strategies.
- The adaptability of organoids means they can be tailored for specific research needs, whether for personalized medicine or high-throughput screening of drugs.
"Organoids stand at the frontier of biomedical research, bridging the gap between simplistic cell cultures and complex in vivo models."
Potential Areas for Future Research
Despite significant advancements, challenges remain in organoid culture protocols. Future research could explore:
- Optimization Techniques: Investigating methods to enhance reproducibility and scalability of organoid cultures, important for broader applications.
- Integration of Biologics: Studying how the incorporation of biomaterials and growth factors affects organoid development and function.
- Technological Innovations: Development of new imaging and analysis tools to monitor organoid behaviors in real-time.
Intro to 3D Organoid Cultures
The study of 3D organoid cultures has grown increasingly important in biomedical research. Organoids, which are miniature, simplified versions of organs produced in vitro, serve as valuable models for understanding development, disease progression, and drug responses. This section aims to highlight the essential elements of 3D organoid cultures, their benefits, and the critical considerations involved in working with them.
3D organoid cultures enable researchers to study cellular interactions in a manner that reflects in vivo conditions more accurately than traditional 2D cultures. This complexity allows for insights into the microenvironment's role in organ development and pathology. Organoids can mimic the architecture and functionality of actual organs, making them a robust platform for various studies.
Definition of Organoids
Organoids are defined as three-dimensional structures derived from stem cells or progenitor cells that self-organize into architectures resembling specific organs. They can reproduce several aspects of tissue function, including cellular organization, gene expression, and the ability to respond to external signals. Common examples of organoids include intestinal, liver, and brain organoids that mimic the structure and function of their respective organs.
These cultures can be created using patient-derived cells, making them ideal for personalized medicine applications. The ability to model diseases with patient-specific organoids offers researchers novel avenues for therapy and insight into disease mechanisms.
Historical Context of Organoid Research
The history of organoid research can be traced back to the early 2000s when scientists began to develop methods to grow stem cells in 3D cultures. The breakthrough came in 2013 when researchers first reported successful protocols for generating intestinal organoids. This approach provided significant advantages over traditional cell culture techniques.
Since then, organoid technology has advanced rapidly. Researchers have expanded the range of organoids generated, including those from organs like the pancreas, lung, and even complex tissues such as the brain. Moreover, the application of organoids in disease modeling and drug testing has revolutionized pharmacological research, providing a more precise context for evaluating therapeutic outcomes.
Organoid technologies are transforming the way researchers approach questions in biology, offering new perspectives on disease modulation, therapeutic discovery, and personalized medicine.
In summary, the field of 3D organoid cultures is monumental for understanding biological systems and advancing medical research, with its historical context underpinning the rapid innovations witnessed today.
Fundamentals of 3D Culture Systems
The relevance of 3D culture systems in the context of organoid research cannot be overstated. These systems emulate in vivo environments more effectively than their two-dimensional counterparts, providing a more accurate representation of cellular behaviors. Cells grown in three-dimensional structures interact in more natural ways, which leads to improved functionality that is crucial for applications such as disease modeling and drug testing.
Moreover, 3D cultures enable the maintenance of tissue architecture that is typically lost in 2D cultures. This characteristic allows researchers to study complex biological interactions, ultimately leading to a deeper understanding of cellular processes.
Overview of 3D Cell Cultures
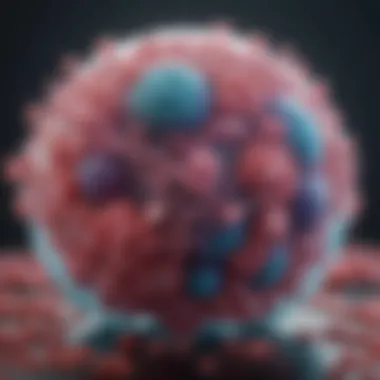
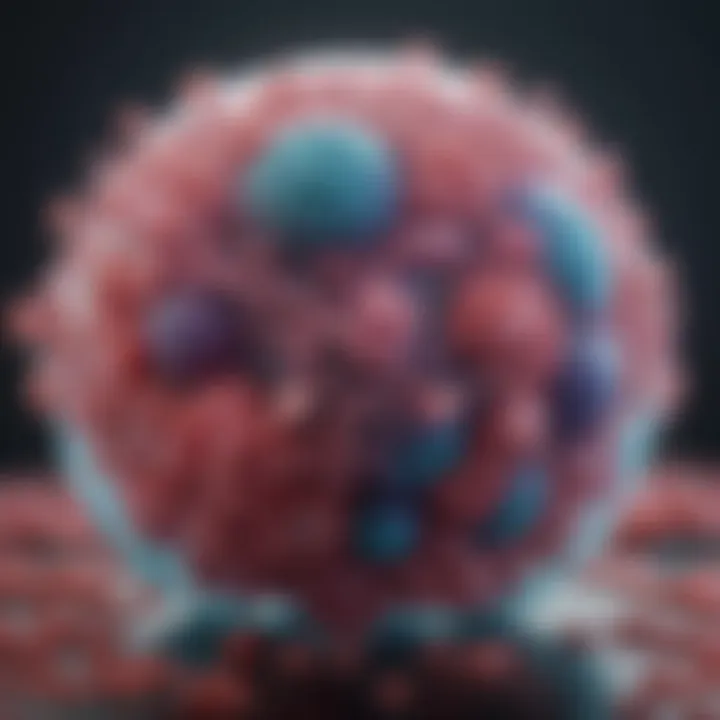
3D cell cultures represent a pivotal advancement in cellular biology, enabling researchers to investigate cell function and behavior in environments that better simulate the natural physiological context. In 3D culture, cells are embedded within a matrix that provides structural support, promoting cell-to-cell interactions essential for maintaining tissue-like properties.
Key advantages of 3D cell cultures include:
- Enhanced cell viability: Cells maintain their function and integrity longer than in 2D systems.
- Realistic responses: Cells exhibit responses to stimuli that are similar to in vivo conditions.
- Increased drug response accuracy: These cultures can predict drug efficacy and toxicity more reliably compared to 2D models.
In applications such as cancer research, 3D cell cultures allow for studying tumor microenvironments and responses to therapeutic interventions, offering insights beyond what traditional methods could provide.
Comparative Analysis: 2D vs. 3D Cultures
In comparing 2D and 3D cultures, it becomes clear that the two systems serve different purposes in research. 2D cultures have long been the standard, but they come with significant limitations. Cells in 2D grow on flat surfaces, leading to altered cell morphology and behavior.
Concerns with 2D cultures include:
- Lack of tissue architecture: Biological functions are not maintained, leading to potential misinterpretation of results.
- Abnormal cellular interactions: Cells do not form the same types of connections as they would in a 3D setting.
- Inconsistent drug responses: Results from drug testing in 2D systems can be misleading due to the lack of a realistic environment.
In contrast, 3D cultures provide:
- Improved mimicry of the in vivo environment: Cells in 3D experience the same microenvironmental cues that they would in a living organism.
- Functional tissue structures: Organoids and other 3D models can exhibit organ-specific functions, enhancing research significance.
- Better representation of disease conditions: Pathological processes are more accurately modeled when cells exist in 3D.
"The adoption of 3D culture systems heralds a new era in biomedical research, with potential to transform our approach to understanding diseases and developing treatments."
Materials Required for Organoid Culture
The effective cultivation of organoids requires a well-defined set of materials. Each component plays a crucial role in ensuring optimal growth conditions for both the cells and the resulting organoids. Understanding the significance of these materials allows researchers to better design their experiments and develop robust protocols.
Cell Sources for Organoid Cultures
The choice of cell sources is fundamental in organoid systems. Organoids can be derived from various tissues, including stem cells, adult progenitor cells, and even differentiated cells. The selection of the appropriate cell type influences not only the growth characteristics of the organoids but also their functional capacity and relevance to disease modeling.
- Embryonic Stem Cells (ESCs): These cells are pluripotent and can give rise to organoids of various tissues. They can form differentiated cell types representing different organs, such as the liver, gut, and brain.
- Induced Pluripotent Stem Cells (iPSCs): iPSCs are another valuable source, as they can be generated from adult cells. This enables the creation of patient-specific organoids, which can be advantageous for personalized medicine.
- Adult Stem Cells: Organoids can also originate from adult tissues, including intestinal and pancreatic stem cells. These cells generally yield organoids that closely mimic the native tissue structure and function, making them suitable for studying specific diseases and conditions.
Choosing the right cell source depends on the intended application. Researchers often weigh factors such as differentiation capacity, availability, and ethical considerations in their decision-making process.
Culture Media: Composition and Selection
The culture medium is pivotal in supporting cell viability and promoting organoid growth. A well-optimized medium is essential for mimicking the in vivo environment that is vital for organoid development. Key components of culture media include:
- Nutrients: Amino acids, vitamins, and sugars are critical for cell metabolism and energy production. The composition should support cellular proliferation and differentiation.
- Growth Factors: Factors like Epidermal Growth Factor (EGF), Fibroblast Growth Factor (FGF), and Wnt proteins provide the stimuli necessary for cell survival and growth. They orchestrate critical signaling pathways that drive organoid morphogenesis.
- Extracellular Matrix Components: Integrating ECM components such as collagen or Matrigel into the medium can enhance cell adhesion and mimic the natural microenvironment. This is critical for maintaining the three-dimensional structure of organoids.
Selecting the right culture medium often requires optimization based on the cell type used. Researchers must perform trials to determine the ideal conditions, as even slight variations in composition can substantially impact organoid growth and functionality.
Matrices and Scaffold Technologies
Matrices and scaffold technologies provide structural support essential for organoid formation and growth. These materials are designed to mimic the extracellular matrix found in living tissues, facilitating better cellular interaction and organization.
- Hydrogels: These are commonly used due to their ability to provide a soft, aqueous environment. Hydrogels can be customized to include growth factors and ECM components, promoting cellular behaviors that resemble native tissue development.
- Decellularized Tissues: Utilized as scaffolds, these matrices come from donor tissues that have been stripped of cellular components, leaving behind a collagen-rich structure. This approach provides a matrix that maintains the natural architecture of the original tissue, offering a conducive environment for organoids.
- 3D Bioprinting: Emerging technologies in bioprinting allow for precise control over the spatial arrangement of cells and extracellular components. This can lead to the creation of highly complex organoid structures, engineered to meet specific research needs.
Step-by-Step Protocol for 3D Organoid Culture
The step-by-step protocol for 3D organoid culture is foundational to ensure success in developing organoids for research. Each protocol comprises defined stages that require precision and optimization to achieve desired outcomes. Understanding these steps is vital, as it influences the viability and function of the organoids produced. There are various considerations, such as the choice of cell type, matrix composition, and specific incubation conditions, which play critical roles in overall effectiveness. Careful adherence to protocol enhances reproducibility and accelerates the translational potential of organoids in biomedical research.
Preparation of Cell Suspension
The preparation of the cell suspension is a crucial first step for successful organoid culture. This process involves isolating and resuspending cells that will be used to form the organoids. This stage requires careful handling to ensure cell viability and maintain their properties. Key aspects to consider include:
- Source of Cells: The cells can originate from primary tissues or established cell lines. Each source has unique characteristics that affect the organoid's behavior.
- Cell Density: The concentration of cells in the suspension must be optimized. Too high or too low density can lead to either clustering too tightly or not forming organoids at all.
- Viability Assay: Using assays like trypan blue exclusion helps assess the percentage of live cells post-isolation, ensuring a good starting point for organoid formation.
Thus, proper preparation directly impacts the quality and functionality of the resulting organoids, serving as the bedrock for further stages.
Embedding Cells in Extracellular Matrix
Embedding cells in the extracellular matrix (ECM) is a defining stage in 3D organoid culture. The ECM serves as a scaffold that mimics the natural environment of tissues, promoting cellular interactions and organization. This step can significantly influence organoid morphology and function. Important considerations include:
- Selection of ECM: Various materials are available, such as Matrigel or collagen, which each provide different properties. The choice can depend on the specific organoid type being cultured.
- Technique of Embedding: Gentle mixing of the cell suspension with the ECM solution before placing it into culture allows for optimal distribution of cells. Techniques like dripping methods or pipetting must be conducted carefully to avoid damaging cells.
- Initial Setting: The ECM must solidify appropriately to offer structural support. Temperature and time parameters should be well defined to ensure the ECM forms correctly.
Embedding is essential as it helps replicate the tissue architecture and microenvironment, leading to functional organoids.
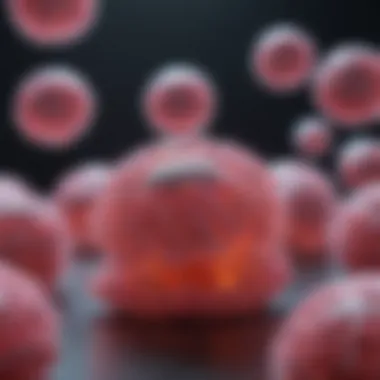
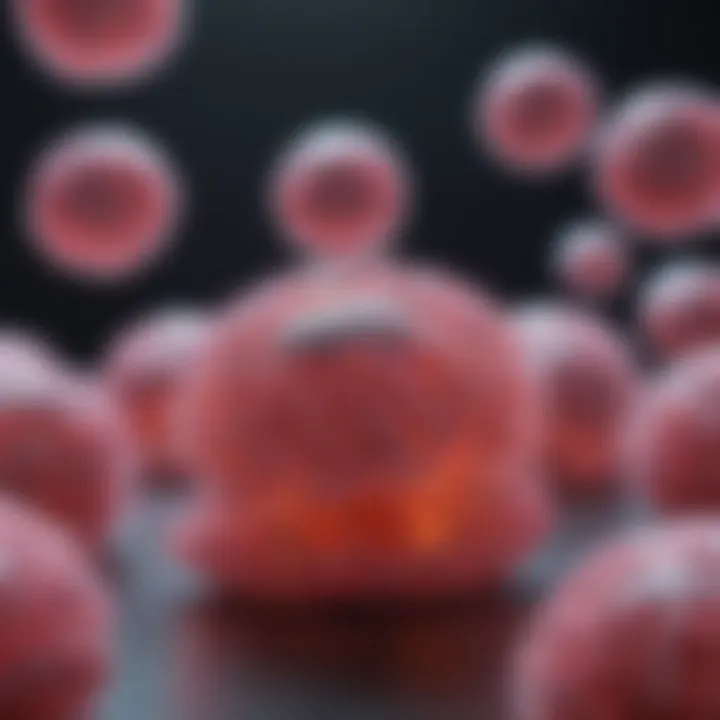
Incubation Conditions
Incubation conditions are critical for the growth and development of organoids. These influence cellular behavior and organoid maturation. Adequately maintaining these conditions shapes many outcome aspects. Factors to consider include:
- Temperature and Atmosphere: Typically, organoid cultures are incubated at 37°C in a 5% carbon dioxide atmosphere. These conditions help to replicate the physiological environment.
- Medium Changes: Regularly changing the culture media helps maintain nutrient availability and remove waste products. This is crucial for prolonged organoid survival and growth.
- Monitoring Growth: Observing organoid development regularly assists in identifying growth patterns and any potential issues requiring intervention.
Proper incubation conditions lead to a higher success rate in organoid formation, making this stage paramount in the overall protocol.
Maintaining precision in each step of the protocol can significantly influence the success and reproducibility of 3D organoid cultures.
Through careful execution and attention to detail at each stage—preparing the cell suspension, embedding in ECM, and maintaining ideal incubation—you shape a promising foundation for producing functional organoids suitable for your research.
Characterization of Organoids
Characterization of organoids is a critical aspect of ensuring their functionality and relevance in research. Organoids, being models that mimic the architecture and physiology of various tissues, require thorough assessment. Characterization allows researchers to understand the developmental stages and biological properties of organoids. This understanding aids in evaluating how well these organoids represent real tissues, which is essential for reliable experimental results.
Effective characterization involves two key aspects: morphological assessment and functional assays. Morphological assessments provide visual and structural information about the organoids, while functional assays evaluate the biological activities. Together, these approaches provide a comprehensive picture of what the organoids can do and how they behave in comparative studies.
Morphological Assessment Techniques
Morphological assessment is foundational for understanding organoid structure and organization. Various imaging techniques can be utilized, including:
- Light microscopy: This method is useful for basic structural visualization. Light microscopy allows for the observation of organoid size, shape, and general architecture. It is often the first step in assessment.
- Fluorescence microscopy: This technique helps visualize specific cellular components using fluorescent markers. It provides insights into cellular organization, potential differentiation, and structural integrity.
- Scanning Electron Microscopy (SEM): SEM offers high-resolution imaging that reveals surface details of organoids. This method is effective for examining microstructural characteristics that influence functionality.
- Confocal microscopy: This is used for detailed optical sections, allowing for 3D reconstructions. It is particularly beneficial for studying internal structures within organoids.
These methods can highlight critical features. For instance, assessing the architecture reveals growth patterns and organization levels. This information is relevant when matching organoid characteristics to those of native tissues.
Functional Assays for Organoid Evaluation
Functional assays are essential for determining the active biological capabilities of organoids. Various functional aspects can be evaluated to understand organoid performance:
- Viability assays: These determine if the cells within organoids are alive and healthy. Techniques like the MTT assay can be employed.
- Metabolic assays: These measure cellular metabolism, which is crucial for understanding organoid functionality.
- Gene expression analysis: Evaluating gene expression profiles helps in understanding the differentiation status and functional attributes of organoids. Both quantitative PCR and RNA sequencing can provide deep insights.
- Drug response assays: Testing how organoids react to various drugs is important for developing therapeutic strategies and understanding disease mechanisms.
Understanding the functional dynamics of organoids is essential for validating their use in research.
By employing both morphological and functional assessments, researchers can optimize organoid cultures for specific applications. Characterization not only verifies the fidelity of organoids but also enhances their utility in biomedical research. This is vital for applications such as disease modeling, drug testing, and personalized medicine.
Challenges in Organoid Culture
The process of culturing 3D organoids is not without its challenges. Understanding these issues is essential, as they can significantly affect the outcome and applicability of organoid research. Recognizing and addressing these challenges is crucial for advancing the field and ensuring that the research is reliable and reproducible.
Variability and Reproducibility Issues
One of the key challenges faced in organoid culture is variability in the cultures themselves. This can arise from a variety of factors including differences in cell source, variations in culture conditions, and the inherent complexity of the organoids.
- Cell Source Variation: The source of cells can influence the phenotype and behavior of the resulting organoids. For instance, organoids derived from different patients or even from different parts of the same tissue can exhibit distinct characteristics. This variability can complicate comparisons across studies.
- Environmental Factors: Conditions such as temperature, humidity, and atmosphere can vary between laboratories. Even small differences in these parameters can lead to considerable changes in organoid development.
- Reproducibility Concerns: Maintaining consistency in experimental procedures is vital. Protocols should be standardized to minimize discrepancies between replicates. Despite best efforts, researchers often find it difficult to reproduce results across multiple experiments.
To counteract these challenges, researchers must implement stringent quality controls and consider using multiple cell lines for validation.
Nutritional Requirements and Maintenance
Nutritional needs of organoids present another significant hurdle. The demands for precise nutrient supply are elevated in a 3D culture compared to traditional 2D systems.
- Complex Media Requirements: Organoids often necessitate specialized culture media that provide specific growth factors and nutrients. The composition of the media may need to be tailored to the type of organoid being cultured, complicating the preparation process.
- Monitoring Nutrient Levels: Routine monitoring and replenishment of nutrients are essential for sustaining organoid viability and functionality. Autonomy in nutrient delivery can lead to over or under-provisioning, both of which can be detrimental.
- Long-term Maintenance: Prolonged culture periods can lead to nutrient depletion and shifts in metabolic states. It's crucial to establish guidelines for regular media changes and nutrient supplementation to ensure optimal growth.
"Proper nutrient supply is essential for the survival and mimicry of organoid tissue characteristics."
Applications of 3D Organoid Cultures
The applications of 3D organoid cultures mark a significant advancement in biomedical research. Organoids have transformed how scientists study diseases, develop drugs, and explore personalized medicine. Each category of organoid application presents distinct benefits that enhance our understanding and treatment of various conditions.
Disease Modeling
Disease modeling through organoids provides an innovative approach to study human diseases in a controlled environment. By mimicking the architecture and function of actual organs, organoids allow researchers to observe disease mechanisms at a cellular level. This is particularly useful for complex diseases such as cancer, where understanding tumor behavior is crucial for therapeutic development.
Unlike traditional 2D cell cultures, organoids can reflect the physiological environment of native tissues. This improvement has profound implications:
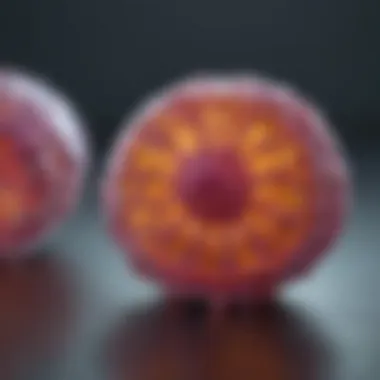
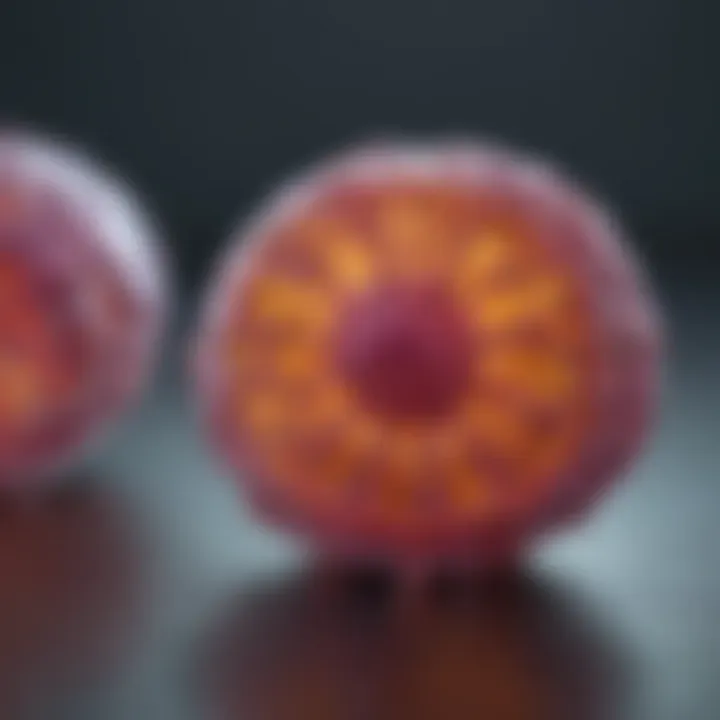
- Pathophysiological Insight: Researchers can investigate how diseases progress and how cells interact within their microenvironments.
- Genetic Variation: Organoids can be derived from patient-specific cells, providing insights into how genetic variations affect disease susceptibility and progression.
- Response to Treatments: Using organoid models allows the testing of various therapeutic options to predict individual patient responses, improving treatment efficacy.
Drug Development and Testing
The development and testing of drugs benefit immensely from the application of 3D organoid culture techniques. Traditional drug testing methods often rely on animal models or 2D cultures, both of which can be limited in their predictive accuracy regarding human responses. Organoids bridge that gap effectively.
Key advantages include:
- High Fidelity: Organoids retain many characteristics of actual tissues, providing a more reliable platform for drug responses compared to conventional models.
- Screening Platforms: Researchers can use organoids to screen large compound libraries efficiently, accelerating the identification of promising drug candidates.
- Toxicity Testing: Assessing the toxicity of potential drugs in organoid cultures helps identify harmful effects early in the development process.
"Organoids are reshaping the landscape of drug development by providing more relevant models for human biology."
Personalized Medicine Perspectives
Personalized medicine is an area poised for significant advancement with the integration of 3D organoid cultures. These models allow for tailored treatment strategies that consider individual patient genetics and disease characteristics. The implications are broad and transformative:
- Patient-Derived Organoids: Organoids can be generated from a patient's own tissue, enabling treatments that are customized to each individual's biological makeup.
- Treatment Response Prediction: By testing potential therapies on patient-derived organoids, clinicians can better predict how a patient’s unique biology will respond to specific treatments.
- Understanding Drug Resistance: Organoids help in studying mechanisms of drug resistance, guiding oncologists in selecting alternative therapeutic strategies for patients who do not respond to standard treatments.
The future of medicine depends increasingly on how well we can tailor therapies to individual needs. 3D organoid cultures are central to that endeavor, promising improved outcomes in various health conditions. These applications not only enhance our understanding of human biology but also pave the way for revolutionary changes in treatment paradigms.
Ethical Considerations in Organoid Research
The ethical dimensions surrounding organoid research demand significant attention. As 3D organoid technology continues to advance, understanding these considerations becomes paramount. Ethical considerations are crucial for fostering trust, ensuring compliance with regulations, and addressing moral responsibilities toward donor tissues.
A cornerstone of ethical discourse involves regulatory guidelines. These guidelines establish the framework within which organoid research should occur. Regulatory bodies have set specific rules to safeguard the interests of donors and to ensure that all research adheres to recognized ethical standards. It is essential to respect the autonomy of individuals providing tissues used in organoid cultures. Compliance with existing laws helps facilitate productive research outcomes while minimizing risks.
"Ethical guidelines not only protect individuals but also promote the integrity of scientific research," - National Institutes of Health.
In the context of organoid research, informed consent and donor tissue use are critical elements. Researchers must obtain explicit consent from donors before using their tissues. This consent must be informed; individuals should be made aware of potential risks, intended uses, and the implications of their contributions. Transparency regarding how tissue will be used in research fosters a sense of agency among donors and can improve participation rates in studies. Moreover, ethical practices encourage the use of tissues that are legally and morally sourced, ensuring dignity and respect for individuals involved.
In summary, ethical considerations in organoid research encompass many facets, from adhering to regulatory guidelines to ensuring informed consent. Navigating these areas with care is crucial for maintaining ethical standards and promoting sustainable practices in the field.
Future Directions in Organoid Technology
The field of organoid technology is rapidly evolving, presenting opportunities to deepen our understanding of complex biological processes. Exploring future directions is essential for maximizing benefits in scientific research, personalized medicine, and therapeutic applications. As researchers enhance current methods and innovate new approaches, the potential to address significant health issues increases. Future advancements will likely focus on improving the accuracy of disease modeling, streamlining drug testing processes, and expanding the types of organoids created. This will involve a combination of interdisciplinary strategies, making it crucial for researchers to stay updated on emerging trends.
Innovative Approaches to Organoid Engineering
Innovating organoid engineering means refining both the methods of creation and the characteristics of the organoids themselves. Here are some potential advancements:
- Bioprinting Techniques: Utilizing 3D bioprinting can lead to more precise spatial organization of cells and scaffolds. This technology allows for the custom design of organoids that mimic biological structures more closely.
- Microfluidics Integration: Incorporating microfluidics enables the dynamic control of the organoid environment. This technology could lead to developments such as organ-on-a-chip systems for enhanced simulation of physiological processes.
- Genetic and Epigenetic Modifications: Utilizing CRISPR and other gene-editing techniques to manipulate organoid genomes could enhance disease modeling capabilities. This approach may allow for studying genetic diseases on a cellular level.
By advancing these techniques, researchers can create organoids that closely resemble in vivo conditions, improving the fidelity of experiments.
Integrating Organoids with Other Technologies
The future of organoid technology may also hinge on its integration with other cutting-edge technologies. This can enhance the applications and functionalities of organoids. Some synergies could include:
- Artificial Intelligence (AI): Leveraging AI to analyze data from organoid experiments can expedite the discovery of patterns and outcomes. Machine learning algorithms can assist in personalizing treatments based on organoid responses.
- Wearable Technology: By combining organoid insights with real-time data from patients, it is possible to develop tailored therapeutic strategies. This integration would allow immediate feedback on treatment efficacy.
- Nanotechnology for Drug Delivery: Using nanoparticles to deliver drugs directly to organoids can enhance the precision of drug testing. This method could lead to more effective therapies with minimized side effects.
Integrating these technologies not only broadens the applications of organoids but also provides richer datasets for research.
"The convergence of multiple technologies is key to advancing organoid research and applications in healthcare."
Continued exploration of future directions in organoid technology is imperative for optimizing their use in research and medicine. As innovative methods emerge, the multifaceted roles that organoids can play in biomedical research will only expand.
End
The conclusion serves as a critical summarizing point in any academic article, encapsulating the key learnings throughout the discussions on 3D organoid culture protocols. This article emphasizes the importance of understanding organoid technology, which is progressively shaping biomedical research. The ability to create organoids from human tissues presents vast potential for advancements in various fields. Through tissue engineering, researchers can simulate organ systems in vitro, paving the way for novel disease models and therapeutic strategies.
Recap of Organoid Significance
In this section, we revisit the pivotal role of organoids in translating research into practical applications. Organoids mimic the architecture and functionality of organs. This feature allows for better model systems compared to traditional 2D cell cultures. Critical insights into cellular behavior are gained without the ethical complications associated with animal research. They provide a robust platform for studying disease mechanisms and drug responses, transforming how drug efficacy and toxicity are evaluated.
To summarize, organoids hold the promise to revolutionize fields such as regenerative medicine and disease modeling. The unique structural and functional characteristics of organoids enhance their relevance, substantiating their emergence as powerful tools in the laboratory.
Call for Continued Research and Development
The field of organoid technology is continually evolving, and there are essential areas that merit further exploration. Continued research is vital for improving protocols that can enhance the yield and consistency of organoid cultures. Standardization of techniques is critical to mitigate variability, a common challenge in organoid research.
Emerging studies focusing on integrating organoids with technologies such as microfluidics and bioprinting can further refine models. Attention should be given to elucidating the mechanisms underlying organoid development, and how these can be applied to more accurately reflect human biology.
As the scientific community embraces organoid culture, there must also be an ongoing dialogue about ethical considerations. Establishing clear guidelines and regulatory frameworks ensures that research advances without compromising ethical standards. Through a multidisciplinary approach, combining ethics, engineering, and biology, a comprehensive understanding and enhancement of 3D organoid cultures can be achieved.