High Resolution Spectrometers: Principles and Innovations
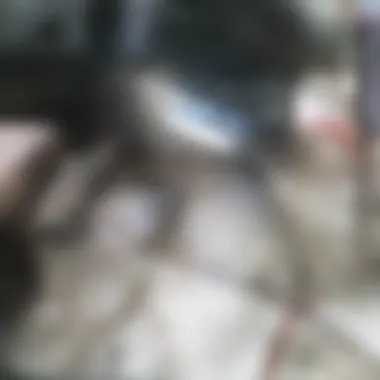
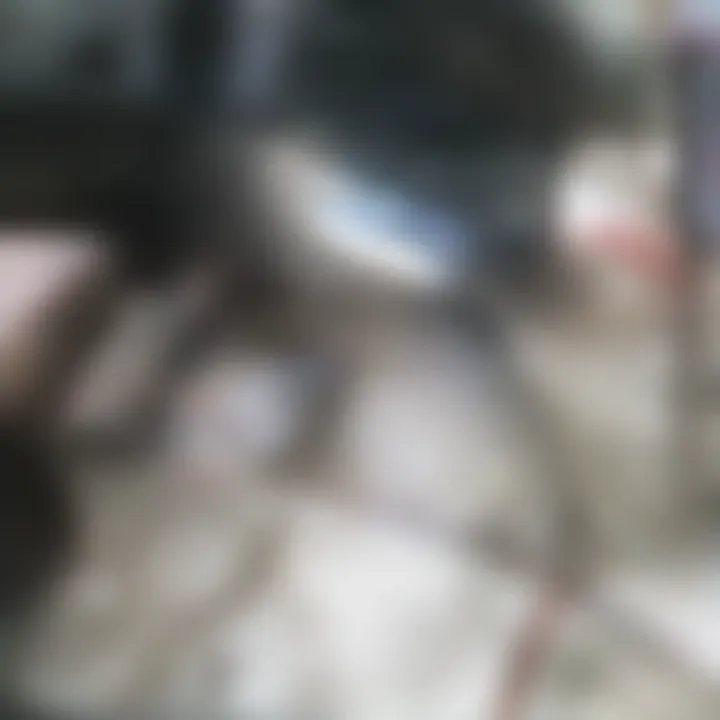
Intro
High resolution spectrometers have become essential tools in scientific research. They allow for precise measurement of various wavelengths of light and have applications across multiple fields such as chemistry, biology, and material science. Understanding how these devices work, their key features, and what innovations are on the horizon is crucial for researchers looking to enhance their experiments and analyses.
This article will provide a comprehensive overview of high resolution spectrometers. From their principles of operation to the technologies that make them versatile, we will delve deeply into their functionalities. Further, we will examine significant advancements in spectrometer technology that are shaping the future of research.
Key Concepts and Terminology
Definition of Key Terms
- Spectrometer: An instrument used to measure properties of light over a specific portion of the electromagnetic spectrum.
- Resolution: The ability of a spectrometer to distinguish between different spectral lines or wavelengths. Higher resolution means finer detail can be discerned.
- Wavelength: The distance between two peaks of a wave, relevant to the colors of light being analyzed.
Concepts Explored in the Article
This article covers several important concepts:
- The basic principles governing the operation of high resolution spectrometers.
- Varieties of spectrometers including Fourier-transform and grating devices.
- Technical specifications that define their performance and capabilities including resolution, range, and sensitivity.
- Practical applications seen in various scientific domains.
- The incremental improvements and innovations enhancing their functionality in research.
The capacity to analyze light with high precision can lead to breakthroughs in material identification and beyond.
Findings and Discussion
Main Findings
High resolution spectrometers play a crucial role in modern science. They facilitate deeper understanding of materials through specific measurements of their spectral fingerprints. Recent advancements have led to more compact designs, faster analysis times, and improved sensitivity. These enhancements have increased their accessibility and usability in laboratories worldwide.
Potential Areas for Future Research
While many advances have been made, the potential for further research remains vast. Some areas that show promise include:
- Integration of artificial intelligence to automate analysis processes.
- Development of portable spectrometers for field studies in environmental science.
- Exploration of new optical materials that could enhance spectral resolution even further.
Prologue to High Resolution Spectrometers
High resolution spectrometers play a pivotal role in advancing scientific research and understanding. These instruments are designed to separate and analyze light into its component wavelengths with remarkable precision. The increase in resolution allows scientists to obtain more detailed information about the substances they are studying.
One significant aspect of high resolution spectrometers is their ability to provide insight into the molecular and atomic structure of materials. This is particularly important for chemists and physicists as they attempt to unravel complex chemical reactions or physical phenomena. By capturing the finer details of a spectrum, researchers can identify minute variations in energy levels and molecular interactions, which can lead to new discoveries and innovations.
The applications of high resolution spectrometers span various disciplines, including chemistry, biology, material science, and environmental monitoring. Some specific benefits of employing these advanced tools include:
- Increased sensitivity: High resolution enables detection of trace elements or compounds that may otherwise go unnoticed in standard measurements.
- Enhanced accuracy: Data collected from high resolution spectrometers tends to produce more reliable and reproducible results.
- Diverse applications: These instruments can be configured for various materials, ensuring their versatility across different research areas.
However, while the advantages are notable, there are considerations researchers must take into account when using high resolution spectrometers. The cost of these instruments can be substantial, which may limit accessibility for smaller labs or less funded projects. Calibration and maintenance are also essential for ensuring data integrity, adding another layer of complexity.
Understanding the principles and methodologies behind high resolution spectrometry is crucial for both emerging scientists and seasoned researchers alike. This article will explore these facets in detail, outlining the technical specifications, types of spectrometers, and their applications across diverse fields.
"High resolution spectrometers illuminate the behavior of matter at molecular and atomic levels, pushing the boundaries of what we know in science."
Fundamental Principles of Spectroscopy
Spectroscopy is a cornerstone of analytical science. Understanding its fundamental principles allows researchers to grasp how high resolution spectrometers operate and their transformative potential in various fields. Spectroscopic techniques form the basis of many scientific inquiries, providing a method to analyze and identify the composition of substances based on their interaction with light. The significance of grasping these principles lies in their utility across multiple disciplines, including chemistry, biology, and physics.
Definition and Basic Concepts
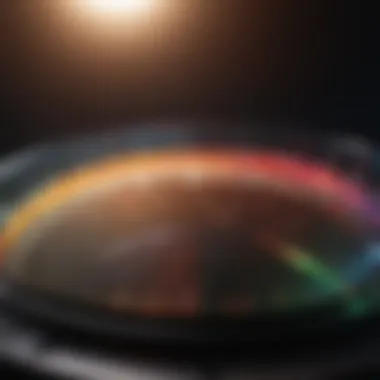
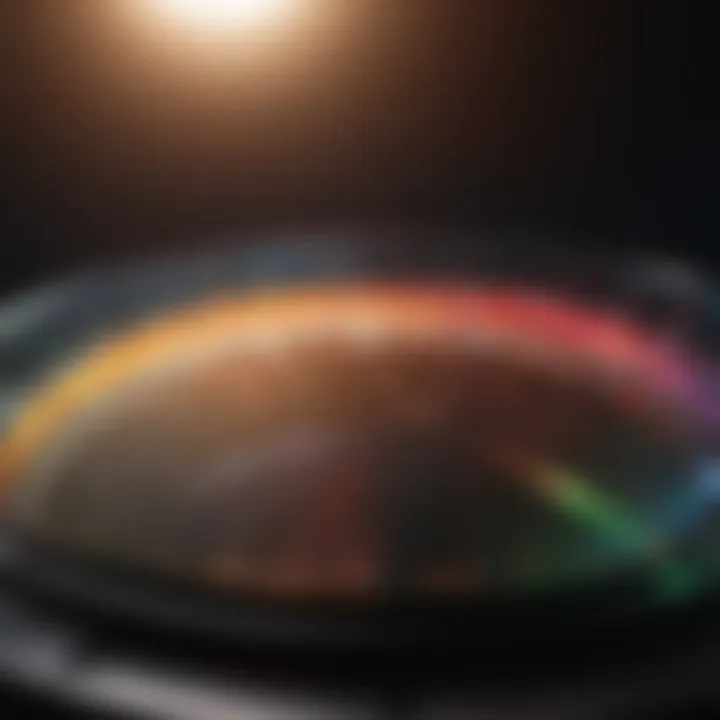
Spectroscopy is defined as the study of the interaction between electromagnetic radiation and matter. This interaction can occur through absorption, emission, or scattering of light. The basic concept revolves around measuring the intensity of light as a function of wavelength or frequency. When a substance interacts with light, it produces a spectrum, which serves as its unique fingerprint. Each material has a distinct spectral signature, allowing for differential identification.
Interaction of Light and Matter
The interaction of light with matter is central to spectroscopy. When light encounters a substance, it can be absorbed by electrons, causing them to transition to higher energy levels. This process leads to the emission of light at different wavelengths when electrons return to their ground state.
Absorption spectroscopy deals with the study of absorbed wavelengths, whereas emission spectroscopy focuses on emitted wavelengths. The energy levels of the electrons in a substance dictate the specific wavelengths that will be absorbed or emitted. This interaction provides insight into the molecular structure and composition of materials. Understanding these interactions can lead to advancements in material science and environmental monitoring.
Importance of Wavelength and Frequency
Wavelength and frequency are crucial parameters in spectroscopy, influencing the resolution and sensitivity of measurements. Wavelength refers to the distance between successive peaks of a wave. In contrast, frequency describes how often these peaks occur in a given time, measured in hertz.
The relationship between frequency and wavelength is given by the equation:
[ c = \lambda f ]
Where:
- (c) is the speed of light,
- (\lambda) is the wavelength,
- (f) is the frequency.
This relationship indicates that when wavelength increases, frequency decreases and vice versa. High resolution spectrometers often work with very fine changes in these properties to detect minor variations in substance composition, leading to better analytical results. Accurate measurement of wavelength and frequency expands the capabilities of spectroscopic techniques, enabling highly precise studies in various scientific domains.
Types of High Resolution Spectrometers
Understanding the types of high resolution spectrometers is crucial for grasping how these instruments function across various applications in scientific fields. Each type of spectrometer serves a unique purpose and offers specific advantages, which are essential for researchers to consider when choosing the appropriate tool for their work. Knowledge of these types allows scientists to maximize resolution, sensitivity, and accuracy in their analyses.
Optical Spectrometers
Optical spectrometers are widely used for their ability to analyze light in different spectral regions. They work by dispersing light into its components using elements like prisms or diffraction gratings, allowing for detailed examination of the wavelength distribution. The key merits of optical spectrometers include their precision and ability to be coupled with modern data acquisition systems, enhancing the accuracy of measurements.
In practical applications, optical spectrometers can be employed in fields ranging from chemistry to environmental science. For instance, they can analyze light absorption of chemical samples, helping in the identification of substances. The development of compact, portable optical spectrometers is also increasing their use in field studies, which is a significant benefit for researchers conducting on-site analyses.
Mass Spectrometers
Mass spectrometers are another significant type of high resolution spectrometer. They operate by ionizing chemical species and sorting the ions based on their mass-to-charge ratios. This capability enables the detection of very low concentrations of substances, making mass spectrometers invaluable in fields such as pharmacology and environmental monitoring.
Key features of mass spectrometers include their high sensitivity and resolution. They can provide insights into complex mixtures, identify unknown compounds, and track biochemical pathways. This makes them a powerful tool in research but also requires careful interpretation of the resulting data, as complexity can lead to challenging analyses.
Nuclear Magnetic Resonance (NMR) Spectrometers
Nuclear Magnetic Resonance (NMR) spectrometers leverage the magnetic properties of atomic nuclei to gather information about the structure of molecules. When placed in a magnetic field, nuclei resonate at specific frequencies depending on their chemical environment. NMR is particularly useful in organic chemistry and biochemistry for determining molecular structures and dynamics.
The interpretability of NMR data is a prime advantage. By providing detailed information on the connectivity of atoms within a molecule, NMR allows researchers to confirm chemical structures accurately. Additionally, advancements in high-field NMR systems have improved sensitivity and resolution, enabling more intricate analyses of complex biological systems.
Vibrational Spectrometers
Vibrational spectrometers, like infrared and Raman spectrometers, focus on the vibrational energy levels of molecules. They provide critical insights into molecular vibrations, which can be correlated to chemical bonding and functional groups. This makes vibrational spectrometers valuable for material characterizations, such as polymer analysis and solid-state investigations.
These spectrometers can be used non-destructively, which is a noteworthy advantage for studying delicate samples. Through techniques such as Fourier-transform infrared spectroscopy (FTIR) or Raman spectroscopy, scientists gain access to qualitative and quantitative information about chemical composition and states without altering the sample integrity.
Vibrational spectroscopy is an essential tool in many laboratories due to its non-destructive nature and ability to provide rich chemical information.
Technical Specifications
Understanding the technical specifications of high resolution spectrometers is vital for researchers and professionals. These specifications directly impact the performance, accuracy, and applicability of the instruments in various fields of study. Key elements include resolution, sensitivity, calibration methods, and data acquisition technologies.
Resolution and Sensitivity
Resolution is a fundamental parameter of spectrometers. It determines how well the device can distinguish between closely spaced spectral lines. High resolution allows for the identification of subtle variations in chemical compositions, which is particularly important in fields like biochemical analysis and material characterization.
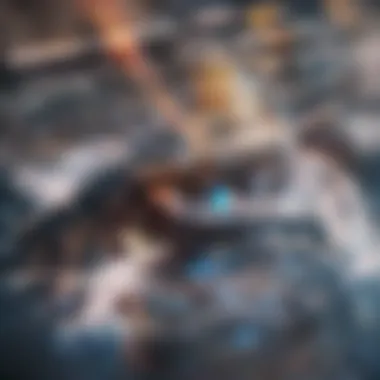
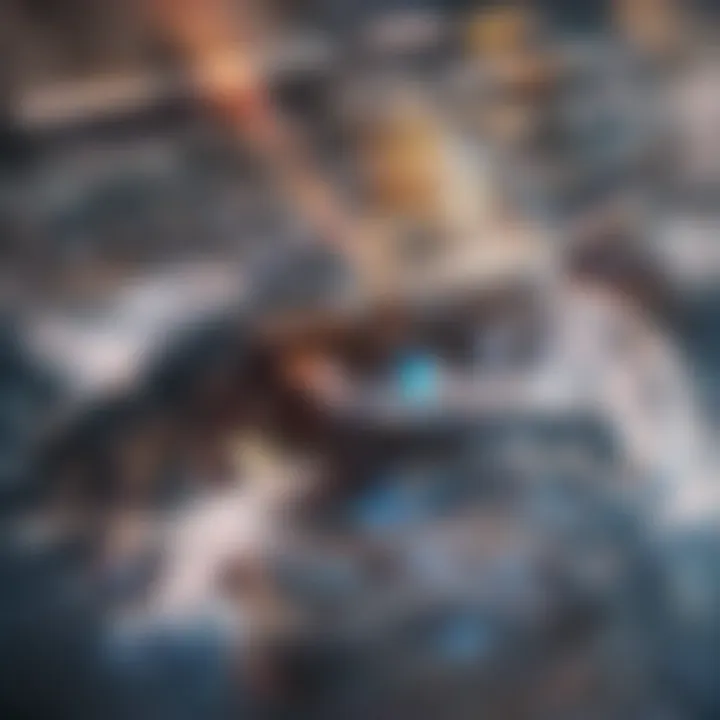
Sensitivity complements resolution, as it refers to the instrument's ability to detect weak signals. A spectrometer with high sensitivity can provide reliable data even from trace samples. This is crucial in applications like environmental monitoring where pollutants may be present in very low concentrations.
Advanced designs and technologies are focused on enhancing both resolution and sensitivity. For example, devices like the Bruker AVANCE NEO offer cutting-edge resolution capabilities, which are essential in complex analyses.
Calibration and Standardization
Calibration ensures that a spectrometer produces accurate and consistent results across different measurements. This process often involves using standards with known properties to align the instrumentβs output with expected values. Regular calibration is essential since measurement accuracy can drift over time due to environmental changes or instrument wear.
Standardization protocols can differ significantly depending on the type of spectrometer and its specific applications. Following established methods, such as using NIST traceable standards, enhances the credibility of data produced.
It's also important for researchers to implement routines for quality control. Frequent checks against standardized readings can prevent costly errors in critical scientific work.
Detectors and Data Acquisition Systems
Detectors transform light or other signals detected by the spectrometer into quantifiable data. The choice of detectors, such as photomultiplier tubes or CCD sensors, influences the performance characteristics of the spectrometer. Each type offers unique advantages, such as speed or sensitivity, which can be tailored to specific applications.
Data acquisition systems play a crucial role in processing the signals registered by the detectors. These systems should efficiently convert raw data into interpretable forms. The integration of advanced software tools permits high speed data analysis, leading to improved accuracy in results. Modern spectrometers, like those manufactured by Ocean Insight, utilize sophisticated software for data analysis and retrieval, significantly enhancing user experience.
Applications Across Disciplines
High resolution spectrometers play a critical role in various fields of science and technology. Their ability to measure and analyze the properties of light allows researchers and professionals to gather valuable data that informs their work. The versatility of these instruments makes them essential in disciplines such as chemistry, biology, material science, and environmental science. Their implementation helps enhance the understanding of complex systems and phenomena.
Chemical Analysis
Chemical analysis is perhaps one of the most significant applications of high resolution spectrometry. These spectrometers provide precise measurements of spectral lines, enabling chemists to identify and quantify substances in a mixture. Techniques like high-performance liquid chromatography (HPLC) and gas chromatography coupled with mass spectrometry (GC-MS) rely heavily on high resolution data to achieve accurate results. This precise identification is crucial for drug development, quality control, and forensic science. Moreover, advancements in spectrometry technology lead to faster analysis times and improved sensitivity, pushing the boundaries of what can be detected at lower concentrations.
Biomedical Research
In the biomedical field, high resolution spectrometers enable researchers to study biological samples at a molecular level. Techniques such as fluorescence spectroscopy and Raman spectroscopy facilitate the examination of biomolecules, providing insights into cellular functions and disease mechanisms. For instance, in cancer research, spectrometry aids in detecting biomarkers that indicate the presence of tumors. These insights are fundamental to developing targeted therapies. Furthermore, the integration of high resolution spectrometry with imaging techniques enhances the understanding of complex biological systems, contributing to the advancement of personalized medicine.
Material Science
High resolution spectrometry has become indispensable in material science, where understanding the properties of materials can lead to breakthroughs in technology and applications. Characterization of new materials, including nanomaterials, polymers, and composites, provides essential information about their structural and functional properties. Techniques such as Fourier-transform infrared spectroscopy (FTIR) allow material scientists to analyze the molecular structure and chemical bonds within solids or liquids. Such capabilities contribute to innovations in fields like electronics, energy storage, and catalysis, significantly influencing material development and performance.
Environmental Monitoring
Environmental monitoring is another vital application of high resolution spectrometry. It helps in detecting pollutants and understanding environmental changes over time. High resolution spectrometers can identify trace amounts of organic and inorganic compounds, providing data critical for assessing air and water quality. For example, the use of spectrometry in monitoring greenhouse gases contributes to climate change research by offering detailed readings of atmospheric composition. Additionally, tracking contaminants in ecosystems helps ensure public health and safety, demonstrating the broader impact of spectrometers in understanding environmental issues.
Advancements in High Resolution Spectrometry
Advancements in high resolution spectrometry hold significant importance in enhancing the capabilities of spectrometers. With the rapid evolution of technology, researchers and professionals can leverage these advancements to obtain more accurate, efficient, and versatile measurement tools. The benefits are numerous and often lead to breakthroughs in various scientific disciplines. Understanding these advancements is key to grasping the future direction of spectrometry.
Technological Innovations
Technological innovations are reshaping the landscape of high resolution spectrometry. These developments focus on improving the precision and range of measurements. For instance, the advancement of ultra-sensitive detectors has increased the ability to detect faint signals in complex samples. This can significantly impact fields such as environmental monitoring, where detecting trace contaminants is essential.
Moreover, innovations in optical components, such as better prisms and gratings, enable scientists to achieve higher resolution without compromising the signal-to-noise ratio. The development of compact spectrometers offers portability without sacrificing performance, which is advantageous for fieldwork and on-site analysis. The integration of fiber optics also allows for remote sensing, expanding the potential applications.
Key benefits of technological innovations include:
- Enhanced sensitivity leading to better detection limits.
- Increased portability for on-site and field studies.
- Improved resolution through advanced optical materials and designs.
- Integration of multi-dimensional data analysis capabilities.
"The continuous evolution in detector technology exemplifies how the field of spectrometry is becoming increasingly adept at meeting diverse scientific challenges."
Integration of AI and Machine Learning
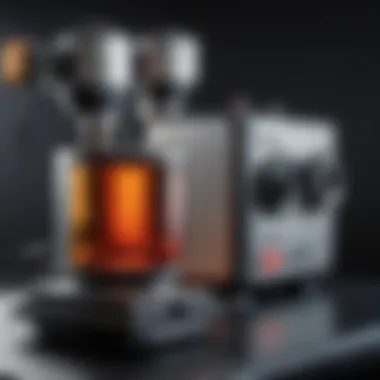
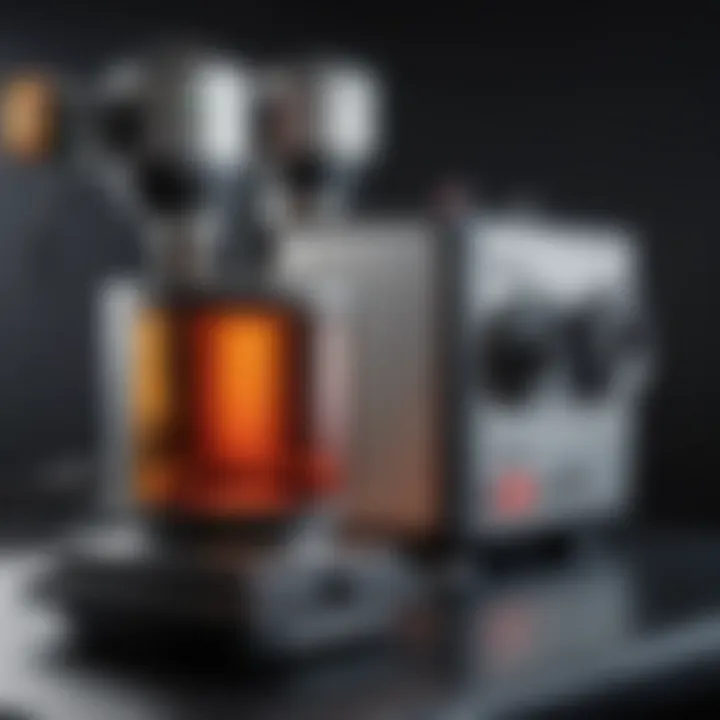
The integration of artificial intelligence (AI) and machine learning (ML) into high resolution spectrometry is a transformative trend. By employing advanced computational techniques, researchers can analyze vast datasets more effectively. AI algorithms have the potential to identify patterns within spectral data that would be challenging and time-consuming for researchers to discern manually.
This integration offers several compelling advantages:
- Automated data analysis: Reduces the time needed for interpretation.
- Predictive modelling: Enhances accuracy in identifying compounds based on spectral signatures.
- Customization: Algorithms can be tailored to suit specific research needs, transforming traditional workflows.
As AI and ML technologies continue to refine, their impact on spectrometry will likely increase, leading to more robust and automated analysis tools. This evolution is crucial for managing the complexity of modern scientific inquiries and fostering interdisciplinary research.
By understanding these advancements, professionals can harness the growing capabilities of high resolution spectrometry, allowing for more informed decisions and innovative approaches in research.
Challenges and Limitations
In the realm of high resolution spectrometry, understanding the challenges and limitations is crucial. This component impacts the practicality and feasibility of employing these sophisticated instruments in various fields. Each issue poses significant considerations that researchers and professionals need to address when utilizing spectrometers.
Cost and Accessibility
One of the foremost challenges in high resolution spectrometry is the cost associated with these sophisticated devices. Spectrometers can represent a substantial investment, often requiring financial resources that some institutions cannot afford. The initial purchase cost, maintenance, and operational expenses add up, making it difficult for smaller laboratories or educational institutions to access this advanced technology.
While grants and funding sources exist, they are often competitive and not guaranteed. As a result, high-quality spectroscopic analysis might be limited to well-funded research centers, leaving a gap in accessibility for those needing the technology. Moreover, intensive user training is needed to operate these instruments effectively, which may add further financial implications in terms of personnel costs.
Data Interpretation and Complexity
The data derived from high resolution spectrometers is another critical aspect posing challenges. The complexity of spectroscopic data requires a high level of expertise in interpretation. Each experimental result can produce an enormous amount of data, which can overwhelm users without the proper analytical skills.
"The interpretation of spectroscopic data is as crucial as obtaining it; errors can easily lead to flawed conclusions."
Analysts must not only understand the basic principles of spectroscopy but also have a firm grasp on statistics and data processing techniques. This expertise is often limited to experienced professionals, leaving novices at a disadvantage. Furthermore, the integration of advanced technologies, such as AI and machine learning, adds another layer of complication, necessitating ongoing education and training for users.
Future Directions in Spectrometry
The field of spectrometry is constantly evolving, driven by technological advancements and the ongoing quest for precision in scientific inquiry. This section explores future directions in this discipline, shedding light on its relevance in comprehensive research environments. The integration of new methods and technologies enhances existing spectrometric capabilities and opens pathways for innovative applications in various scientific disciplines.
Emerging Trends
Recent advancements in spectrometry focus on enhancing resolution and sensitivity. Technologies like high-resolution mass spectrometry are gaining traction in laboratories. Next-generation optical spectrometers now utilize better detectors, which improve signal-to-noise ratios, enabling analysis of minute quantities of substances.
Moreover, miniaturized spectrometers, such as those used in smartphones, illustrate a trend towards portable solutions. These instruments democratize access to spectrometric tools, allowing more scientists to engage in sophisticated analysis without the necessary budget for large, traditional setups.
Another significant trend is the incorporation of quantum technologies. Quantum sensors offer unprecedented sensitivity and accuracy, promising opportunities to detect previously unattainable signals. This reflects a broader convergence of classical spectrometry with cutting-edge quantum informatics, expanding the frontier of what is possible in molecular analysis.
"The future of spectrometry will hinge on the versatility of tools and methods available to researchers, paving ways for innovation across disciplines."
Potential for Cross-disciplinary Applications
Spectrometry is not confined to a single field; its future leans towards multi-disciplinary applications. For instance, collaboration between chemists and biologists can create biological sensors that monitor cellular processes in real-time. This integration significantly enhances research in biotechnology.
Additionally, chemical industry professionals are finding utility in combining spectrometry with machine learning. Utilizing large datasets generated from spectrometric analyses aids in predictive modeling, hence refining product development processes.
Environmental science also stands to benefit from cross-disciplinary approaches. Techniques developed for chemical analysis can be implemented for ecological monitoring, tracking pollutants in various contexts. This illustrates how advancements in spectrometry can serve broader societal needs, emphasizing sustainability and health.
End
The conclusion serves as a final reflection on the significance of high resolution spectrometers and their indispensable role in various scientific inquiries. Throughout this article, we have explored the principles, applications, and advancements related to these sophisticated instruments. High resolution spectrometers provide precise measurement capabilities that are crucial for an accurate analysis of materials and phenomena.
One of the core benefits lies in their ability to discern minute differences in spectral lines. This precision is especially important in fields such as biomedical research, where subtle changes in molecular structures can indicate the presence of diseases or the effectiveness of treatments. The integration of advanced technologies further enhances their application across disciplines, expanding the horizons of traditional spectrometric methods.
Considerations about the future trajectories of spectrometry reveal a landscape rich with potential. The emerging trends, such as the incorporation of artificial intelligence and machine learning, promise to revolutionize data analysis and interpretation. Moreover, as spectrometers become more accessible, a broader segment of researchers and professionals may leverage these technologies to drive innovation.
In summary, the evolution of high resolution spectrometers not only enhances our understanding of the microscopic world but also opens avenues for interdisciplinary collaboration, fostering a spirit of inquiry that can lead to transformative discoveries.
The journey of exploring high resolution spectrometers emphasizes the need for continual learning and adaptation in scientific methodologies. The insights gained from their advancements equip researchers to tackle complex problems, making the study of these instruments vital for future explorations in science.