Exploring Microbial Whole Genome Sequencing Techniques
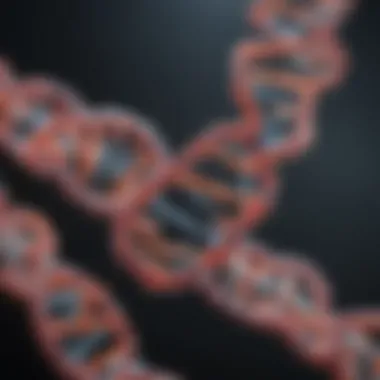
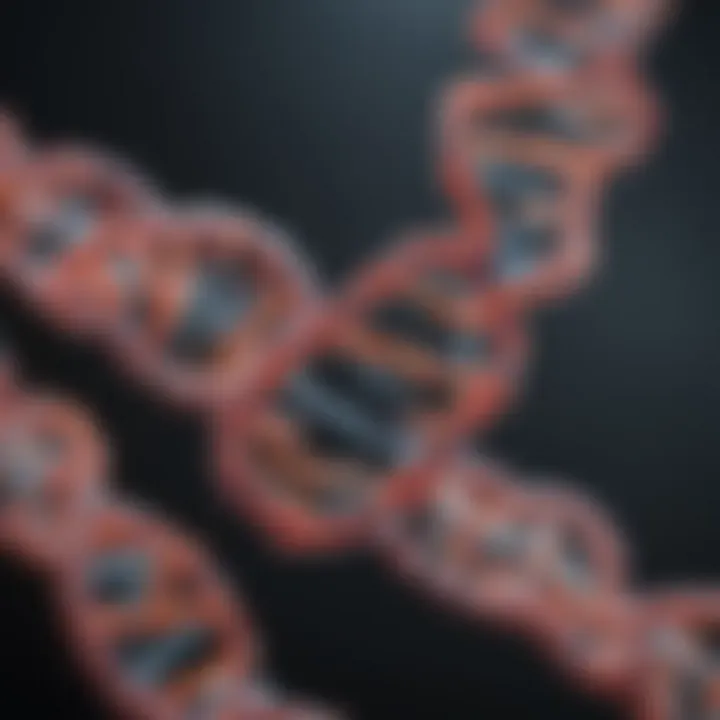
Intro
Microbial whole genome sequencing (WGS) has emerged as a pivotal tool in modern biological research. This technique enables scientists to decode the entirety of microbial DNA, providing comprehensive insights into genetic makeup and function. With the capacity to manage complex datasets, researchers can explore a vast array of applications across diverse fields โ from identifying pathogens in medicine to understanding ecological interactions in environmental science.
The transition from traditional methods to WGS marks a significant leap in our ability to investigate microbial life. This article will serve as a guide through the essential aspects of microbial WGS, discussing key concepts, its methodology, practical applications, and the challenges faced in this evolving landscape.
Key Concepts and Terminology
Definition of Key Terms
Understanding microbial WGS requires familiarity with several key terms:
- Genome: The complete set of genetic material within an organism.
- Sequencing: The process of determining the order of nucleotides in a DNA molecule.
- Microbial Diversity: Refers to the variety of different microbial species present in an environment.
- Pathogenicity: The ability of an organism to cause disease.
- Antimicrobial Resistance: The ability of microbes to resist the effects of medications that once killed them.
Concepts Explored in the Article
Throughout this article, several important concepts will be covered:
- The methodology of WGS and various sequencing technologies such as Illumina and Nanopore.
- Applications of WGS in identifying pathogens, studying microbial communities, and monitoring environmental health.
- Current challenges, particularly around data analysis, interpretation, and ethical concerns.
- Future prospects for advancements in sequencing technology and its implications for research.
Findings and Discussion
Main Findings
The advent of WGS has revolutionized the way researchers view and study microorganisms. Some key findings include:
- Enhanced Pathogen Detection: WGS allows for precise identification of pathogenic organisms, crucial for diagnosing infectious diseases.
- Comprehensive Microbial Analysis: Researchers can now explore complex microbial communities, uncovering roles of different species in health and disease.
- Understanding Resistance Mechanisms: With detailed genomic data, scientists can study how microbes develop resistance to antimicrobials, aiding in the development of new treatment strategies.
Potential Areas for Future Research
Several promising directions for future research in microbial WGS are emerging:
- Integration with Metagenomics: Combining WGS with metagenomic approaches could enhance the understanding of microbial ecosystems.
- Real-time Sequencing Applications: Developing faster sequencing technologies could pave the way for rapid pathogen identification during outbreaks.
- Exploration of the Human Microbiome: Further studies on how microbial genomes interact with human health can provide new insights into disease prevention and treatment.
"Microbial WGS is more than just a method; it represents a paradigm shift in our understanding of life at the microscopic level."
As we advance, it is crucial to address the challenges associated with microbial WGS. Effective data management and ethical considerations will become increasingly vital as the community harnesses this powerful tool for scientific progress.
Prolusion to Microbial Whole Genome Sequencing
Microbial whole genome sequencing (WGS) is an evolving and crucial area in genomics that merits attention for its profound implications in various scientific fields. Its significance lies in the power it grants researchers to access detailed information about the genetic material of microorganisms. Understanding these genetic sequences is essential for unraveling the complexities of microbial diversity, pathogenicity, and resistance mechanisms. In a world increasingly impacted by microbial infections and environmental challenges, the importance of effective WGS cannot be overstated.
The ability to sequence entire genomes allows scientists to gain a deeper insight into how microbes interact with their environments. It also allows for the identification of specific strains and their potential impacts on public health. The knowledge gained from microbial WGS can inform clinical diagnostics, track disease outbreaks, and contribute to advancements in biotechnology.
Considering the aforementioned attributes, this article will present a comprehensive exploration of microbial whole genome sequencing, highlighting its methodology, applications, challenges, and future perspectives. This is intended primarily for students, researchers, educators, and professionals who seek in-depth understanding and practical knowledge in this domain.
Definition and Overview
Microbial whole genome sequencing defines the comprehensive sequencing of the entire genome of microorganisms. This includes not just the genes, but also non-coding regions that may have regulatory roles. Whole genome sequencing can provide insights into various genomic features, such as genetic diversity, phylogenetic relationships, and evolutionary patterns.
WGS leverages several sequencing technologies. These technologies allow for the complete reconstruction of an organism's genetic profile, which is pivotal in fields such as medical microbiology and environmental science. In essence, microbial WGS is a vital tool for researchers aiming to explore the genetic basis of microbial life.
Historical Context
The journey of microbial whole genome sequencing traces back to the early days of genetic research. With the advent of DNA sequencing technologies in the 1970s, scientists began to unravel the structure of genetic materials. The pioneering work of Frederick Sanger introduced Sanger sequencing, which dominated for decades. However, as microbial genomics increasingly gained traction, the limits of Sanger sequencing became evident due to its time-consuming and costly nature.
In the early 2000s, the sequencing of the Haemophilus influenzae genome marked an important milestone, as it was the first free-living organism to have its genome fully sequenced. This achievement showcased the potential of WGS in understanding microbial genomes.
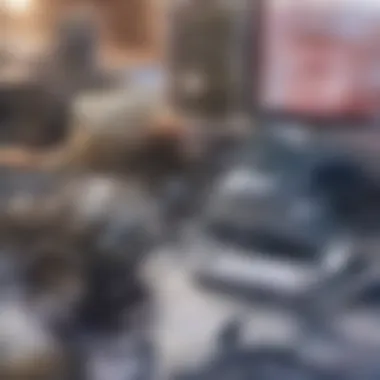
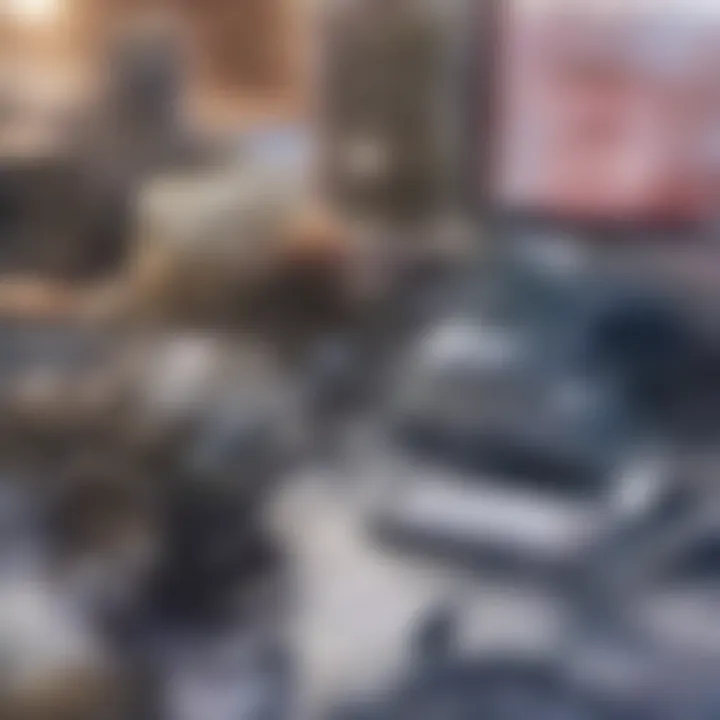
The evolution continued with the advent of next-generation sequencing technologies, which revolutionized the ability to sequence genomes at unprecedented speed and reduced costs. This progress paved the way for a more extensive exploration of microbial diversity and genomes, making microbial WGS a cornerstone in modern microbiological research.
The combining of historical advancements with modern technological trends positions microbial whole genome sequencing as an indispensable element in scientific inquiry, paving paths for future discoveries about microorganisms.
The Methodology of Whole Genome Sequencing
The methodology of Whole Genome Sequencing (WGS) is crucial in microbiology, as it forms the backbone of how researchers analyze the genetic makeup of microorganisms. Understanding the different sequencing techniques available allows researchers to select the most suitable one for their specific needs. In the realm of microbial WGS, the methodology not only improves the accuracy of identification but also provides insights into the genomic features of pathogens and beneficial microbes alike. Each stage, from sample preparation to data interpretation, holds distinct importance. A thorough understanding of these elements can lead to significant advancements in various applications, including medical diagnostics, environmental studies, and agricultural biotechnology.
Overview of Sequencing Techniques
Sanger Sequencing
Sanger sequencing is one of the earliest techniques used for DNA sequencing. It is based on the principle of chain termination, where modified nucleotides create fragments of varying lengths during replication. This method is highly accurate and can sequence short DNA fragments with great precision. Sanger sequencing is particularly beneficial for projects requiring detailed DNA analysis, such as verifying sequences obtained from more high-throughput methods. However, its limitations in scalability and time efficiency make it less favorable for larger genomes or metagenomic studies.
Next-Generation Sequencing (NGS)
Next-Generation Sequencing represents a significant leap in sequencing technology. Unlike Sanger sequencing, NGS allows for the simultaneous sequencing of millions of fragments, dramatically increasing throughput. NGS is affordable and efficient, enabling the examination of entire microbial communities rather than just individual samples. This technology has become popular because of its versatility and capacity to produce vast amounts of data quickly. Despite its advantages, the complexity of data analysis and potential for higher error rates in homopolymeric regions are notable drawbacks.
Third-Generation Sequencing
Third-Generation Sequencing differs from both Sanger and NGS by allowing for the sequencing of single molecules of DNA. Techniques like Pacific Biosciences' SMRT sequencing utilize long-read technology, enabling researchers to capture entire genomic regions in one go. This is crucial for resolving complex repetitive sequences and structural variants that shorter-read methods may miss. The ability to generate longer reads offers the possibility of assembling more accurate genomes. However, it remains relatively more expensive compared to NGS, and the throughput may not always meet the demands of large-scale projects.
Sample Preparation and DNA Extraction
Sample preparation and DNA extraction are foundational steps that significantly impact the quality of sequencing results. A well-extracted DNA sample is essential for obtaining comprehensive sequencing data. Various methods exist for DNA extraction, including mechanical, enzymatic, and chemical approaches. Each has its own advantages and challenges.
Data Analysis and Interpretation
Data analysis is where the raw sequences are transformed into meaningful information. This involves several steps, including bioinformatics tools, genome assembly, and annotation techniques.
Bioinformatics Tools
Bioinformatics tools play a pivotal role in analyzing sequences obtained from WGS. These tools can process large datasets, aligning sequences, and helping to identify variations. Tools like Galaxy and QIIME have gained recognition for their user-friendly interfaces and extensive functionalities. Their substantial contribution allows researchers to discern genetic features efficiently. However, learning to use these tools can require a steep learning curve.
Genome Assembly
Genome assembly involves reconstructing a microbial genome from the sequences obtained. It merges overlapping DNA fragments into a continuous sequence. The process can be categorized into different strategies, such as de novo assembly and reference-based assembly. Each method has distinct strengths. De novo assembly is essential when no reference genome exists. However, the accuracy of the assembled genome can vary due to sequencing errors.
Annotation Techniques
Annotation techniques are essential for assigning functional information to genes identified in the sequences. This involves the use of databases and software tools to predict gene functions and annotate genomic features. Accurate annotation is critical for translating raw sequence data into insights about microbial functionality. Yet, the accuracy of annotations can be hindered by incomplete databases and the ever-evolving nature of microbial genomics.
Proper methodology in microbial WGS not only enhances the accuracy of identification but also reveals deeper biological insights.
Applications of Microbial WGS
Microbial whole genome sequencing (WGS) has emerged as a crucial tool in contemporary microbiology. The applications of this technology extend across various domains, significantly enhancing our capability to understand microbial behaviors, interactions, and their implications in health and ecology. Each application reveals unique benefits and limitations, contributing collectively to the broader goals of research and practical advancements.
Clinical Diagnostics
Pathogen Identification
Pathogen identification is a pivotal aspect of clinical diagnostics utilizing microbial WGS. By analyzing genetic sequences, it allows for the accurate identification of pathogens causing infections. This capability is vital for implementing effective treatment regimens rapidly. One of the key characteristics of this approach is its high sensitivity and specificity compared to traditional methods. This ensures that even obscure or less common microorganisms can be detected.
Moreover, the unique feature of pathogen identification through WGS is its ability to differentiate between closely related species or strains. This can help healthcare professionals tailor antibiotic therapy effectively, enhancing patient outcomes. However, a significant drawback is the need for robust bioinformatics infrastructure and trained personnel to interpret the complex data generated.
Antimicrobial Resistance Profiling
Antimicrobial resistance profiling is another critical application of WGS in clinical settings. This methodology involves assessing the genetic basis of resistance mechanisms in pathogens. This provides insights into the treatment possibilities and helps predict treatment outcomes. A notable aspect of resistance profiling is its ability to monitor emerging resistance trends in bacterial populations.
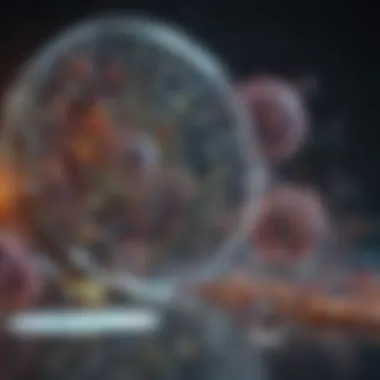
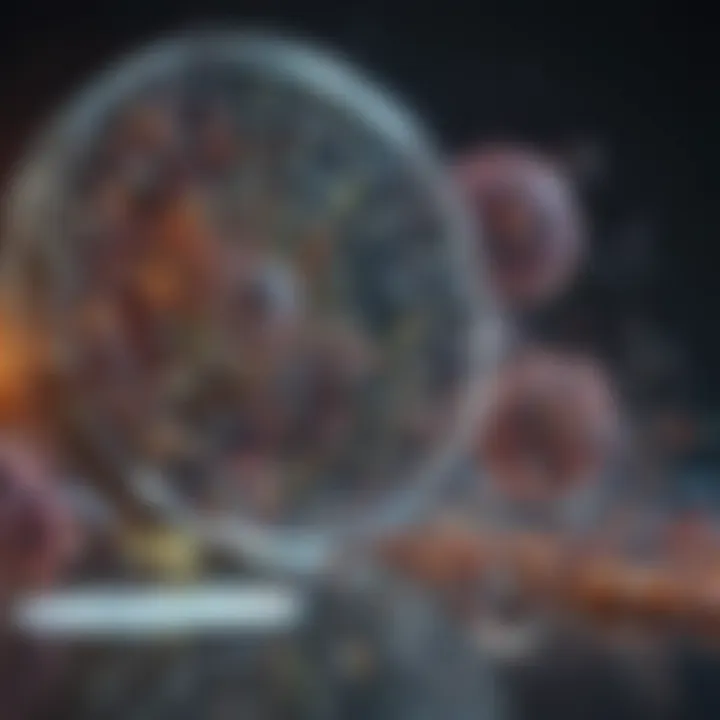
This application is especially beneficial because it helps inform public health strategies aimed at controlling the spread of resistant strains. The unique feature here is the speed at which data can be generated, allowing for real-time surveillance of resistance patterns. Nevertheless, challenges persist in terms of standardizing the methods and ensuring accessibility of the data across different healthcare systems.
Epidemiology and Outbreak Investigation
In the realm of epidemiology, microbial WGS plays a vital role in outbreak investigations. It provides tools to track the source of infections and monitor transmission pathways. Using genomic data, researchers can establish evolutionary relationships between strains, assisting in identifying how outbreaks occur. This application enhances our understanding of disease dynamics in populations, allowing for more effective interventions.
The real-time analysis afforded by WGS facilitates quicker response times to emerging public health threats. However, the need for extensive databases and geographical data is a limitation that must be addressed for optimal outcomes in this application.
Environmental Microbiology
Bioremediation
Bioremediation represents a practical application of microbial WGS that focuses on cleaning contaminated environments using microorganisms. This technique highlights the potential of specific microbial communities to degrade pollutants like heavy metals or organic compounds. A major characteristic of bioremediation is its environmentally friendly approach compared to traditional chemical methods.
The unique feature of using WGS in bioremediation is the ability to identify metabolic pathways utilized by microorganisms. This can enhance the design and implementation of bioremediation strategies. However, the variability of microbial communities in different environments poses challenges in predicting outcomes and effectiveness.
Microbial Ecology Studies
Microbial ecology studies benefit from WGS, enabling a more profound understanding of biological interactions in various ecosystems. By revealing the richness and diversity of microbial communities, researchers can understand how these organisms interact with their environment. A key characteristic of this application is its capacity to provide data on previously uncharacterized species.
The unique feature of employing WGS here revolves around metagenomic approaches, allowing for comprehensive assessments of microbiomes. However, data complexity and interpretation remain significant challenges in translating these findings into applicable knowledge for environmental management.
Agricultural Biotechnology
Soil Microbiome Analysis
Soil microbiome analysis is essential for understanding the health and fertility of soils in agricultural contexts. WGS allows researchers to explore the diverse microbial inhabitants of soil and their roles in nutrient cycling. This is crucial for sustainable agricultural practices. A central characteristic of this analysis is the precision with which it can identify key microbial taxa associated with soil health.
One unique benefit of soil microbiome analysis is its potential to tailor agricultural practices based on the specific microbial profiles present. Yet, a disadvantage is the complexity of soil ecosystems, which can complicate the direct application of findings in practical farming scenarios.
Plant-Microbe Interactions
Plant-microbe interactions encompass a significant area of research using microbial WGS. This involves exploring how microbes impact plant growth, health, and productivity. A notable characteristic of this application is its relevance in developing biofertilizers and biopesticides. These products can enhance agricultural resilience against environmental stressors.
The unique feature of investigating these interactions is the insight it provides into symbiotic relationships that can optimize crop yields. However, the specificity of plant-microbe relationships can limit the broader applicability of findings across different species and ecosystems.
Microbial WGS serves as a gateway to deeper insights into the microbiological world, with applications shaping future research directions and practical solutions across fields.
Challenges in Microbial Whole Genome Sequencing
Microbial whole genome sequencing (WGS) brings forth valuable insights into the genetics of microorganisms, yet it is not without its complexities. Understanding the challenges associated with this technology is essential for advancing research and applications in various fields. The following sections delve into the specific challenges, outlining their implications and significance.
Technical Limitations
Coverage Gaps
Coverage gaps are a notable concern in microbial whole genome sequencing. These gaps refer to regions of the genome that are either underrepresented or entirely absent in the sequence data. This issue can arise due to the complexities involved in sequencing different types of microbial genomes, especially those that are large or contain repeat sequences. One key characteristic of coverage gaps is that they can hinder accurate genome assembly and annotation. Consequently, this introduces uncertainties in functional interpretations and hinders our understanding of microbial characteristics and behaviors. Moreover, coverage gaps can lead to misidentifications or incomplete insights into the evolutionary relationships among different microbial strains. Thus, addressing coverage gaps remains crucial for enhancing the reliability and effectiveness of WGS.
Error Rates in Sequencing
Error rates in sequencing pose another significant challenge in microbial WGS. These errors can occur during the sequencing process, leading to incorrect base calls, which ultimately affect the fidelity of the genetic data generated. A high error rate can compromise the integrity of the sequencing results, making it difficult to draw accurate conclusions about the microorganism of interest. It is important to acknowledge that different sequencing platforms exhibit varying error characteristics, often influenced by the technology employed. While some techniques may offer higher throughput, they might also introduce a higher frequency of errors. Therefore, selecting appropriate sequencing methods and implementing effective error correction strategies are vital steps in ensuring the accuracy and usability of WGS data.
Data Management Issues
Data Storage
Data storage is a critical aspect in the realm of microbial whole genome sequencing. The amount of data generated by WGS can be colossal, requiring robust and scalable storage solutions. With the advent of Next-Generation Sequencing technologies, the volume of genomic data continues to swell, leading to potential bottlenecks in data storage capabilities. One important attribute of data storage is that it must facilitate not only the retention of extensive datasets but also provide accessibility for analysis. Additionally, various storage formats contribute to storage complexity. Researchers often face difficulties in managing diverse dataset types, creating challenges in effective data retrieval and analysis processes. Striking a balance between storage capacity and accessibility is a constant pursuit in the world of microbial genomics.
Data Sharing and Accessibility
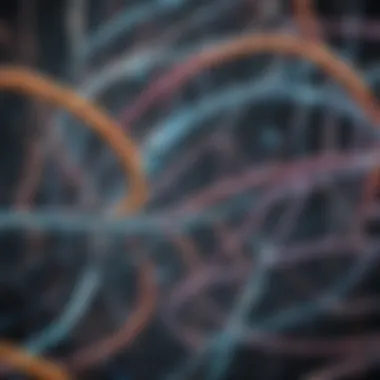
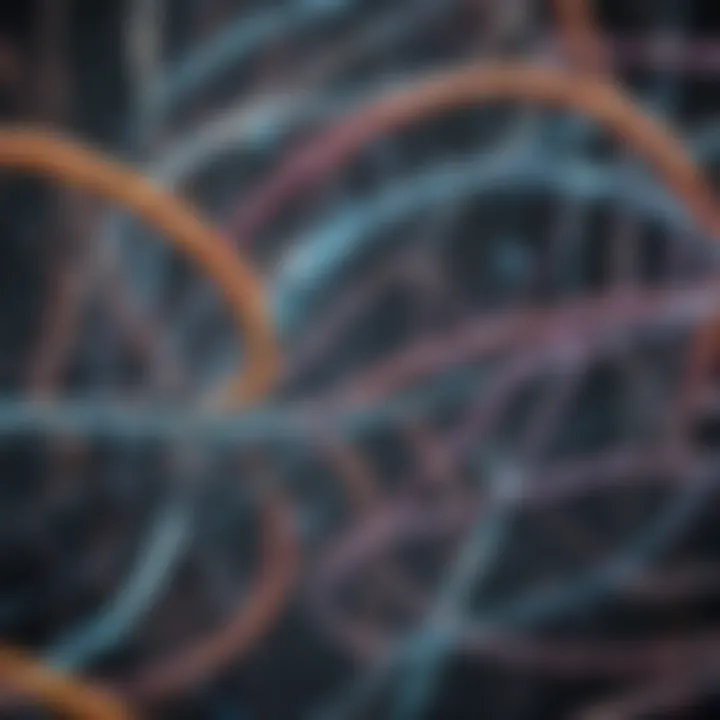
Data sharing and accessibility are paramount for fostering collaboration and advancing research in microbial WGS. However, several obstacles hinder seamless data sharing practices, including differences in data formats, variations in technological capabilities, and concerns over privacy and data ownership. A significant feature of this challenge is that researchers often struggle to find effective mechanisms to share their findings and access data generated by others. This can lead to duplication of efforts and a slower pace of scientific progress. Establishing universal standards for data sharing can elevate the collective understanding of microbial genomics and enhance collaborative research initiatives. Without the ability to share data effectively, the benefits of WGS may be limited.
Ethical and Regulatory Considerations
The ethical landscape surrounding microbial whole genome sequencing cannot be overlooked. Issues related to privacy, consent, and data ownership create a complex web of considerations that researchers must navigate. Questions about how microbial genomic data can be used, who owns the data, and the implications for public health also arise. Particular attention should be paid to the ethical treatment of data, especially in cases where human-associated microbiomes are involved. Additionally, regulatory frameworks surrounding the usage of WGS data can influence research directions and priorities. Adhering to ethical guidelines ensures that the advancements made in microbial genomics respect individual rights and promote responsible research endeavors.
Navigating the challenges of microbial whole genome sequencing requires a multifaceted approach that addresses technical, management, and ethical issues. By recognizing and tackling these challenges head-on, researchers can unlock the full potential of this powerful technology.
The Future of Microbial WGS
The future of microbial whole genome sequencing (WGS) is poised to reshape how we approach a variety of fields within biology and medicine. As technology evolves, its applications will expand, leading to new insights and innovations. Understanding the significance of these developments is crucial for researchers keen on maximizing the potential of WGS.
Emerging Technologies
Advancements in Sequencing Techniques
Advancements in sequencing techniques represent a significant leap forward for microbial WGS. One of the most important aspects is their increased speed and accuracy. As techniques improve, the time required to sequence genomes decreases significantly. This brings about more rapid responses in clinical diagnostics and research. Next-gen sequencing, for instance, is a popular choice. It allows for massive data generation at lower costs, promoting widespread adoption. Its key characteristic lies in its ability to sequence millions of fragments simultaneously. This enhances throughput and efficiency in data collection.
However, despite its advantages, there are limitations. Error rates might still pose a challenge, albeit less than older methods. Hence, understanding these methodologies is essential for effective implementation.
Real-Time Sequencing
Real-time sequencing is another promising direction for the future of microbial WGS. This technique provides immediate information on sequences as they happen, facilitating faster analysis. Its primary characteristic is the ability to conduct sequencing in a portable format. This can be particularly beneficial in field settings or for on-site diagnostics. Real-time sequencing reduces the time frame from sample collection to actionable results, which is critical in outbreaks or emergencies.
Yet, this method does require robust computational resources for analyzing streaming data. The advantages in rapid diagnostics come with the need for enhanced data management capabilities.
Integrative Approaches
Metagenomics
Metagenomics allows for studying genetic material recovered directly from environmental samples. It contributes to microbial WGS by providing insights into community structures and functions without needing isolated cultures. This technique stands out due to its ability to offer a more comprehensive view of microbial diversity. It focuses on the genetic microbes in their natural habitats. Such a method is beneficial as it overcomes the limitations of traditional culturing techniques, which often miss uncultured microorganisms.
However, analyzing metagenomic data can be complex. The diversity of genetic material in a sample complicates the assembly and interpretation processes. Thus, specific computational tools are needed to manage this complexity effectively.
Functional Genomics
Functional genomics plays a vital role in complementing microbial WGS. This approach allows researchers to analyze the roles of various genes and their interactions. It can reveal how specific genes contribute to functions, such as microbial survival and resistance mechanisms. A notable aspect of functional genomics is its ability to connect genotype to phenotype, providing a clearer picture of how specific genetic variations influence behavior.
Though functional genomics is powerful, it also comes with challenges. Analyzing data requires sophisticated bioinformatics methods and a deep understanding of gene functions. Therefore, while this approach contributes significant value, handling its complexities will require dedicated resources.
Potential Impacts on Public Health
As the future of microbial WGS evolves, its implications for public health cannot be overlooked. The role of WGS in tracking infectious diseases, understanding resistance patterns, and monitoring environmental health is profound. It opens possibilities for targeted interventions, thereby improving overall health outcomes. Bridging the gap between sequencing technology and public health practices will further enhance disease prevention and control strategies. Researchers, policymakers, and public health officials must collaborate to leverage the full potential of this technology in combating health issues related to microbial pathogens.
End
The conclusion of this article serves as a vital synthesis of the expansive subject of microbial whole genome sequencing (WGS). WGS has evolved into an indispensable technology in various fields, enabling detailed insights into microbial genetics. From clinical diagnostics to environmental microbiology, its applications are extensive and continue to expand.
WGS offers several key benefits. First, it provides comprehensive data that uncovers the complexities of microbial genomes. This understanding is crucial for identifying pathogens in clinical settings. Secondly, it supports epidemiological studies, aiding in outbreak investigations. The depth of information available through WGS not only facilitates accurate identification but also informs strategies for managing public health crises.
Relevant ethical considerations are essential to address as WGS technology progresses. With the potential for significant impacts on privacy and data sharing, it is vital that researchers and practitioners establish strict guidelines. The future of WGS hinges not only on technological advancements but also on responsible use and management of the information gleaned from microbial genomes.
Summary of Key Points
- Definition and Importance: Microbial WGS allows for an in-depth analysis of complete genetic material, vital for studying pathogenicity, resistance, and diversity.
- Methodological Advances: Various techniques, including Next-Generation Sequencing, enhance the efficacy of genomic studies.
- Applications: Clinical diagnostics and outbreak investigations can greatly benefit from WGS, leading to improved public health responses.
- Emerging Technologies: Innovations in sequencing technologies hold promise for real-time analyses and integrative approaches to studies.
- Ethical Considerations: Issues related to data management and privacy must be addressed as WGS technologies advance.
Final Thoughts on WGS Implications
Microbial whole genome sequencing stands at the forefront of modern biological research and health care. Its implications reach wide and far, catalyzing progress in various scientific domains. As technology advances, we anticipate that WGS will facilitate a greater understanding of complex microbial interactions, evolution, and environmental adaptations.
While the benefits are immense, the considerations surrounding data ethics and accessibility require ongoing discourse. These discussions are crucial for harnessing the potential benefits while mitigating risks associated with genetic data. Ultimately, the future of microbial WGS will not only reflect technological advancements but also our collective responsibility to manage and utilize this powerful resource judiciously.
"Microbial whole genome sequencing is a transformative approach that reshapes our understanding of microorganisms and their roles in health and disease."
This summary solidifies the potential for WGS to revolutionize scientific inquiry and public health strategies. The focus on ethics and responsibility will ensure that this powerful tool is used wisely for the benefit of society and the environment.