Exploring the Nerve Complex: Structure and Function
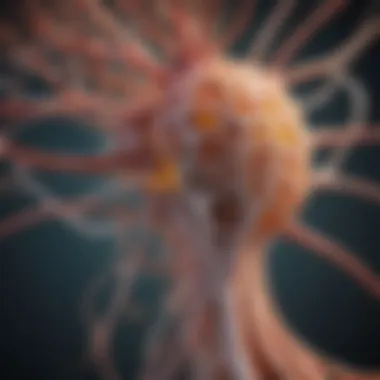
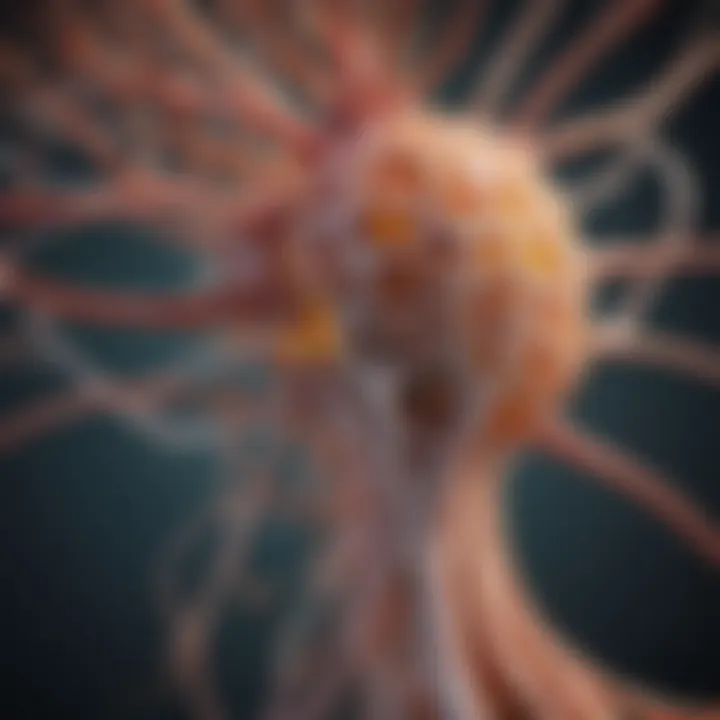
Intro
Nerve complexes are essential structures within the nervous system that play a crucial role in facilitating communication and processing sensory information. Understanding the framework of these complexes providesvaluable insights into how organisms interact with their environments. This section sets the stage for a comprehensive examination of nerve complexes, their significance, and the broader implications for biological systems.
Key Concepts and Terminology
Definition of Key Terms
To fully grasp the intricacies of nerve complexes, it is important to clarify a few key terms. Here are some definitions:
- Nerve Complex: A network of interconnected nerve cells that transmit signals throughout the nervous system.
- Neurons: The primary cells in the nervous system responsible for transmitting information.
- Synapses: The junctions between neurons that allow for signal transmission.
- Neurotransmitters: Chemical messengers that facilitate communication between neurons.
- Sensory Processing: The mechanism by which sensory information is interpreted and understood by the nervous system.
Concepts Explored in the Article
This article explores several critical concepts relating to nerve complexes, including:
- The structural organization of nerve complexes and how this impacts their functionality.
- The role of nerve complexes in sensory processing and the response mechanisms to environmental stimuli.
- The recent advancements in research examining nerve complexes, focusing on their clinical implications and therapeutic potential.
Findings and Discussion
Main Findings
The exploration of nerve complexes reveals several important findings:
- Structural Complexity: Nerve complexes exhibit a highly organized structure, with neurons intricately connected through synapses, forming pathways that are vital for effective communication.
- Functional Significance: Nerve complexes are integral to sensory processing. They enable organisms to detect and respond to changes in their environment, contributing to survival and adaptation.
- Interconnectedness: Individual nerve complexes do not function in isolation. They influence and are influenced by surrounding complexes, creating a vast and interdependent network that is essential for the overall functionality of the nervous system.
Potential Areas for Future Research
Future research could delve into the following areas:
- Regenerative Medicine: Investigating how nerve complexes can be repaired or regenerated in cases of injury could provide therapeutic options for individuals suffering from nerve damage.
- Neurodegenerative Diseases: Understanding how alterations in nerve complexes contribute to diseases such as Alzheimer’s or Parkinson’s could yield insights into new treatment methodologies.
- Technological Advancements: The impact of technologies such as neuroprosthetics and brain-computer interfaces on the functionality of nerve complexes is an emerging area for exploration.
Nerve complexes are not just a network of connections; they are dynamic entities that continually adapt and respond to internal and external stimuli.
Intro to Nerve Complexes
Nerve complexes represent an intricate network fundamental to understanding neural functions. They encompass a variety of elements, including neurons and glial cells, which work in tandem to transmit signals, process data, and maintain overall brain health. The structural organization of these complexes is critical for their functionality, shaping how information flows within the nervous system. In this section, we will delve into the definitions and historical context that paved the way for modern neuroscience. These foundational concepts will set the stage for a more detailed exploration in subsequent sections.
Defining Nerve Complexes
Nerve complexes are multi-layered systems that include neurons, which are the primary signaling units, and glial cells, which support and protect these neurons. Neurons transmit information through electrical impulses and chemical signals. Glial cells provide essential services such as nutrient transport, waste removal, and insulation through myelination. Understanding nerve complexes requires recognizing them as both a structural and functional unit of the nervous system. Their interaction allows for complex communication processes, enabling organisms to respond to stimuli and interact with their surroundings effectively.
Historical Context
The study of nerve complexes has evolved significantly over time. Early investigations by scientists like Santiago Ramón y Cajal laid the groundwork for our understanding of neuron structures and functions. His work established the neuron doctrine, which states that neurons are the basic building blocks of the nervous system. Later research expanded upon these ideas, incorporating glial cells and their roles in nervous system function. Today, researchers employ advanced imaging techniques and molecular biology tools to explore the complexities of nerve networks in unprecedented detail. This historical foundation highlights the progressive nature of neuroscience, emphasizing how contemporary studies build on previous knowledge.
"The history of neuroscience is marked by breakthroughs that continue to influence our understanding of the nervous system today."
Through this exploration of nerve complexes, we aim to illuminate the complexities of neural communication, as well as their broader implications on biological systems. This foundational understanding is vital for grasping the more detailed discussions on anatomy, physiology, and clinical significance that follow in the article.
Anatomy of Nerve Complexes
The anatomy of nerve complexes holds significant relevance in understanding the intricate workings of the nervous system. This section provides insight into the components that make up nerve complexes, emphasizing the relationship between structure and function. Understanding the anatomy allows researchers and professionals to appreciate how these structures contribute to essential processes like sensory input, motor coordination, and cognitive function. The complexity of neural anatomy serves not only to enhance our knowledge but also aids in developing therapeutic interventions and research methodologies.
Neurons and Glial Cells
Neurons are the primary functional units of the nervous system. They are specialized cells responsible for transmitting signals. Each neuron consists of a cell body, axons, and dendrites. The axon transmits impulses away from the cell body, while dendrites receive signals from other neurons. Neurons operate through a process called action potentials, which transmit electrical signals rapidly across long distances.
Glial cells, on the other hand, support and protect neurons. They are numerous and include several types such as astrocytes, oligodendrocytes, and microglia.
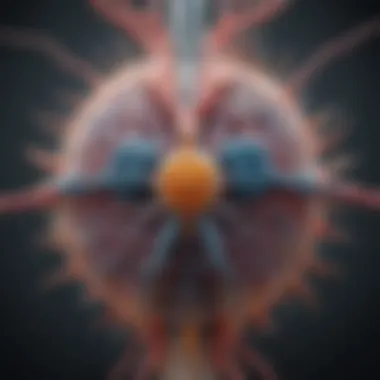
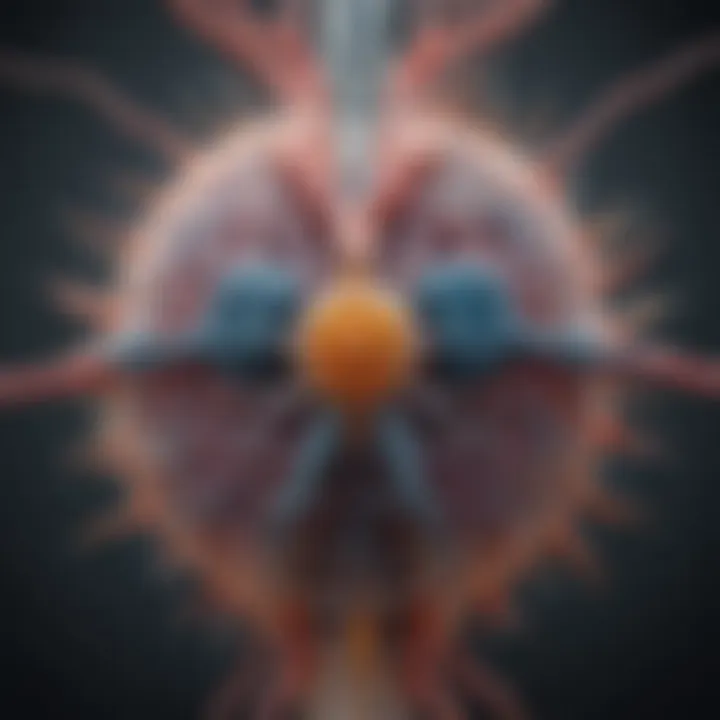
- Astrocytes regulate the environment around neurons, ensuring they receive adequate nutrients.
- Oligodendrocytes form the myelin sheath, crucial for quick signal transmission.
- Microglia function as the immune cells of the nervous system, responding to injuries and infections.
Understanding the roles of these cells enhances our grasp of how the nervous system functions holistically and how disturbances in cell activity may lead to neurological disorders.
Synaptic Structures
Synapses are the junctions where communication between neurons occurs. They are vital for neural signaling. At the synapse, neurotransmitters are released from the presynaptic neuron and bind to receptors on the postsynaptic neuron. This process can be excitatory or inhibitory, influencing whether the next neuron will transmit an action potential.
Several key structural components make up synapses:
- Presynaptic membrane: Contains vesicles filled with neurotransmitters.
- Synaptic cleft: The gap between the two neurons.
- Postsynaptic membrane: Houses receptors that detect neurotransmitters.
The precise arrangement of these structures is crucial for effective communication between neurons. Synaptic plasticity, the ability of synapses to strengthen or weaken over time, also plays an important role in learning and memory.
Nerve Pathways and Networks
Nerve pathways are channels along which signals travel within the nervous system. They are composed of multiple interconnected neurons, allowing efficient communication across different regions of the brain and body.
These pathways can be categorically divided into:
- Ascending pathways: Carry sensory information to the brain.
- Descending pathways: Transmit motor commands from the brain to the body.
The integration of nerve networks allows for complex behaviors and responses to stimuli. The organization of these pathways is a subject of ongoing research, as understanding these connections can illuminate how brain injuries or disorders affect overall function. Advanced imaging techniques are proving useful for mapping these networks, which improves our knowledge of both normal and pathological states.
Physiology of Nerve Complexes
The physiology of nerve complexes is crucial for understanding how information is processed in the nervous system. This topic offers insight into the mechanisms that underlie signal transmission, influencing everything from reflex actions to complex behaviors. Understanding these mechanisms can lead to advancements in treating neurological disorders and enhancing technologies such as neural interfaces.
Action Potentials
Action potentials are the fundamental units of electrical signaling within neurons. They occur when a neuron is activated, causing rapid changes in membrane potential. This change propagates along the axon, allowing for the transmission of signals over long distances. The all-or-nothing nature of action potentials ensures that once a certain threshold is reached, the signal will continue to its destination without diminishing.
Factors influencing action potential generation include:
- Threshold potential: The level of depolarization required to trigger an action potential.
- Ion channels: Sodium and potassium channels play key roles in depolarization and repolarization.
- Axon diameter: Larger diameters increase conduction velocity due to decreased resistance.
Neurotransmission Mechanisms
Neurotransmission is the process by which neurons communicate with each other. This process involves the release of neurotransmitters from presynaptic neurons and their binding to receptors on postsynaptic neurons. Various types of neurotransmitters, such as serotonin, dopamine, and glutamate, have distinct roles in modulating neural activity and influencing behaviors.
Key aspects of neurotransmission include:
- Vesicle fusion: Neurotransmitters are stored in vesicles and released into the synapse upon arrival of an action potential.
- Receptor specificity: Receptors vary in their sensitivity and response to different neurotransmitters.
- Reuptake processes: After binding, neurotransmitters can be reabsorbed into the presynaptic neuron, terminating the signal.
Regulation of Nerve Activity
Regulation of nerve activity ensures that the nervous system responds appropriately to both internal and external stimuli. This regulation occurs at multiple levels, including synaptic modulation and feedback loops involving higher brain areas.
Factors to consider include:
- Inhibitory and excitatory signals: Balance between these signals can influence overall neural activity.
- Neuroplasticity: The ability of neural connections to strengthen or weaken over time affects learning and memory.
- Hormonal influences: Hormones can modify nerve activity, impacting mood and behavior.
"The interplay of action potentials, neurotransmission, and activity regulation is fundamental to the nervous system's operation, making the physiology of nerve complexes essential to both basic and applied neuroscience."
Understanding the physiology of nerve complexes provides foundational knowledge for various fields, including medicine, psychology, and computer science. Each aspect discussed plays a critical role in how organisms interact with their environments and adapt to changes.
Functions of Nerve Complexes
Understanding the functions of nerve complexes is crucial in grasping their role within biological systems. These complexes facilitate communication throughout the body, impacting not only how we respond to the environment but also how we process information. By examining specific elements such as sensory input processing, motor coordination, and cognitive and emotional responses, it becomes clear just how vital these nerve structures are for daily functioning and overall health. The implications of disruptions within these systems extend into various fields such as medicine, psychology, and even technology.
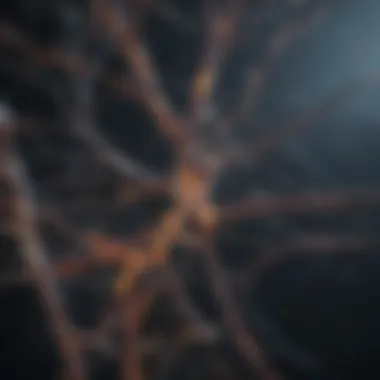
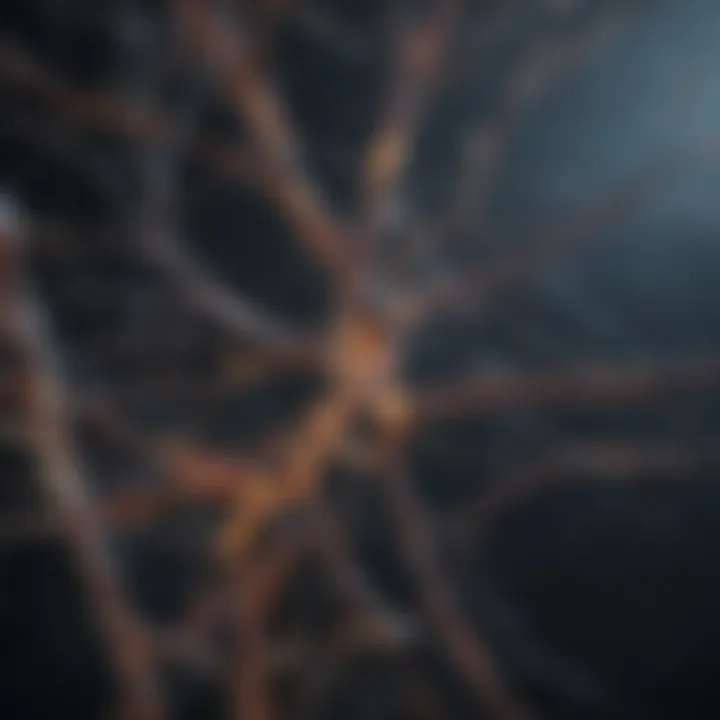
Sensory Input Processing
Nerve complexes are fundamental in the processing of sensory input. They receive stimuli from the environment through sensory neurons, which convert physical phenomena into electrical signals. These signals travel through various pathways in the nervous system and reach specific regions of the brain, where interpretation occurs. The complexity of this system allows for a nuanced understanding of sensations like sight, sound, and touch.
The role of glial cells in this processing cannot be underestimated. They support neurons and help maintain the homeostasis necessary for efficient signal transmission. Furthermore, the involvement of neurotransmitters in synaptic transmission ensures that sensory information is relayed quickly and accurately. Disruptions in any part of this complex can lead to sensory processing disorders, demonstrating how tightly knit these structures are in function.
Motor Coordination
Motor coordination involves the integration of sensory information with motor commands to produce smooth and precise movements. Nerve complexes coordinate this entire process by connecting sensory inputs with the motor outputs via complex neural pathways. The cerebellum plays a pivotal role in ensuring balance and refinement of movements, while spinal cord reflexes provide rapid reactions to stimuli without requiring higher brain involvement.
Essentially, when the brain sends signals to muscles, it relies on nerve complexes to execute those instructions effectively. A well-coordinated neuromuscular system allows for activities ranging from simple actions like grasping an object to complex motions such as playing a musical instrument.
Cognitive and Emotional Response
Beyond motor coordination, nerve complexes significantly influence cognitive and emotional responses. They enable processes such as learning, memory, and emotional regulation. Neural circuits in regions of the brain, such as the prefrontal cortex and limbic system, are responsible for these higher functions.
The interplay between different neurotransmitters, such as dopamine and serotonin, greatly affects mood and behavior. Understanding this can lead to insights in treating psychiatric conditions. Moreover, disruptions in these circuits can result in cognitive impairments, affecting how individuals process information and interact with their surroundings.
"The capabilities of nerve complexes highlight their centrality in integrating sensory experiences, adjusting movements accordingly, and shaping cognitive and emotional landscapes."
In summary, the functions of nerve complexes encompass a broad spectrum of activities essential for interaction with the environment. From sensory processing to motor control and cognitive functions, these complex systems play an integral role in human behavior and health. Their study offers insights that can influence various fields, from neuroscience research to clinical applications.
Clinical Significance of Nerve Complexes
Understanding the clinical significance of nerve complexes is fundamental in both medical practice and research. These structures are critical in maintaining and regulating the physiological functions of the nervous system. They are involved directly in pathways related to health and disease, making their study essential for improving therapeutic outcomes.
Nerve Injuries and Repair
Nerve injuries can have profound effects on function. They may arise from trauma, compression, or disease. Significant losses in sensory, motor, or autonomic functions may occur, resulting in pain and disability. Depending on the severity of the injury, the nervous system may have a limited ability to repair itself.
Repair processes involve several key mechanisms:
- Wallerian Degeneration: This refers to the process that follows nerve injury where the axon segment distal to the injury site degenerates.
- Axonal Regeneration: The surviving nerve fibers attempt to reconnect with their target tissues.
Challenges exist due to the complexity of nerve anatomy. Repair methods include surgical interventions, nerve grafts, and even biotechnological methods such as growth factors.
Neurological Disorders
Neurological disorders often stem from disruptions in nerve complexes. Conditions such as Alzheimer's disease, multiple sclerosis, and epilepsy highlight how imperfections in nerve communication can lead to severe implications. The functioning of nerve complexes may involve:
- Dysregulated neurotransmitter release: This affects cognitive and physical functions.
- Neuroinflammation: Inflammatory responses can lead to damage of nerve cells across various diseases.
Research continues to reveal the interplay between specific nerve complexes in different conditions, paving way for targeted treatment strategies. Understanding these paths allows for identification of potential therapeutic targets and drug development.
Therapeutic Interventions
Various interventions target nerve complexes in hopes to restore function or alleviate symptoms associated with nerve injuries or disorders. Some prominent methods include:
- Medication: Drugs like anticonvulsants for epilepsy or anti-inflammatory agents for neuroinflammation.
- Physical Rehabilitation: Physical therapy can enhance recovery through systematic exercises.
- Surgical Options: Reconstructive surgeries and neurostimulation devices are being explored to improve outcomes in chronic pain and motor function recovery.
The dynamic nature of research offers hope for advancements in repairing and strengthening nerve complexes. It is essential to monitor developments in treatment strategies to optimize patient care.
"The emerging fields of neuroregeneration and neurobiology offer potential breakthroughs that could vastly improve quality of life for those affected by nerve-related issues."
Monitoring new studies and findings improve knowledge and application of these treatments. Continuing to explore this area is invaluable. The clinical significance of nerve complexes extends well beyond the laboratory; it has real world implications that affect therapy and wellness.
Current Research in Nerve Complexes
Contemporary research into nerve complexes represents a critical area of study within neuroscience and related disciplines. Analyzing recent findings aids in understanding how these structures contribute to the brain's adaptability, especially in response to experiences. The website en.wikipedia.org explains this growing field extensively, detailing research initiatives that focus on brain function. By prioritizing current data, researchers are revealing novel insights about neuroplasticity, neural networks, and their implications for future studies.
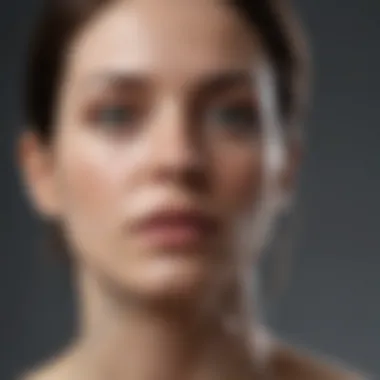
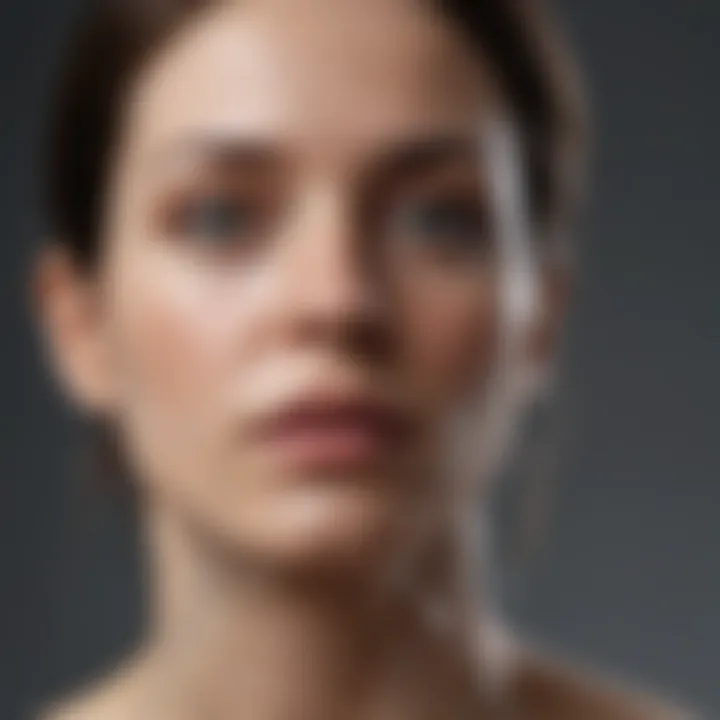
Advances in Neuroplasticity
Neuroplasticity, the ability of the brain to reorganize itself, is a central theme in current research on nerve complexes. This adaptability allows for recovery from injuries and the development of new skills. Recent findings suggest that exercises and specific therapies can significantly enhance one's ability to learn. In patients with neurological disorders, for example, engaging in targeted activities promotes rehabilitation. The exploration of how nerve pathways evolve offers vital knowledge for various therapeutic approaches.
- Key considerations in neuroplasticity research:
- Mechanisms underlying synaptic change
- Role of neurotrophic factors in nerve growth
- Impact of environmental factors on neural connectivity
Understanding these aspects can foster better intervention strategies in clinical settings, especially for individuals dealing with trauma or developmental issues.
Investigating Neural Networks
Research into neural networks emphasizes how interconnected neurons function together, especially in complex tasks. This investigation reveals how the brain analyzes information, thus optimizing cognitive processes. Techniques like functional MRI have advanced our ability to visualize these networks in real time. Such studies highlight how distributed networks underlie various functions, from basic motor skills to higher-order thinking.
"Understanding neural networks provides insights into the collective behavior of neurons, influencing technology and artificial intelligence development."
The implications of these discoveries extend beyond neuroscience, influencing fields like psychology, robotics, and artificial intelligence. This cross-disciplinary approach deepens understanding of how complex systems operate.
Future Directions in Nerve Complex Studies
Looking ahead, future research in nerve complexes aims to uncover deeper insights into their role within the broader neurological landscape. Areas such as chronic pain, aging, and degenerative diseases are likely to benefit from ongoing inquiries. Researchers focus on innovative methods to assess nerve health, including high-resolution imaging and biotechnological tools.
Focus points in future research include:
- Investigating cellular mechanisms at a molecular level
- Understanding the implications of cross-modal neuroplasticity
- Developing advanced therapeutic techniques, such as gene therapy
As these studies progress, the integrative nature of nerve complexes will become clearer. This could lead to breakthroughs in treating neurodegenerative diseases, enhancing cognitive function, and improving overall mental health.
Interdisciplinary Perspectives
The examination of nerve complexes is not confined to the boundaries of neuroscience alone. It is an interdisciplinary endeavor that intersects with various fields, each contributing unique insights and tools necessary for a holistic understanding of these intricate systems. The interplay between biology, psychology, and technology creates a richer narrative about the roles and implications of nerve complexes in both health and disease.
Nerve Complexes in Physiology
In physiology, the study of nerve complexes is crucial for understanding the mechanics of the nervous system. These complexes consist of neurons and glial cells, anatomy that relates to functional processes within the body. The interaction among different cell types provides vitality to functions like synaptic transmission. Adequately functioning nerve complexes are essential for maintaining homeostasis and facilitating communication between different systems. An understanding of the physiological underpinnings equips researchers and healthcare professionals with the knowledge needed to diagnose and treat conditions linked to nerve dysfunction effectively.
Influence on Behavioral Sciences
Behavioral sciences benefit greatly from insights gained in the study of nerve complexes. The relationship between neural activities and behavior is profound; cognitive processes, emotions, and even decision-making stem from the functioning of these networks. Research into neurotransmission and synapse function sheds light on how changes in nerve complexes can lead to alterations in behavior and mental health disorders. The study of nerve complexes thus aids in developing interventions that can modify behavior, making it a valuable asset for psychologists and counselors working with patients.
Integration with Technology
Technological advancements have revolutionized the understanding of nerve complexes. Tools like functional MRI and electrophysiological recordings allow for real-time monitoring of nerve activity. This integration has not only fostered innovation in research but has also highlighted the potential for developing neuroprosthetics and brain-computer interfaces. The collaboration between neuroscientists and engineers exemplifies how technology can unravel complex biological questions. Such integration creates opportunities for translating insights into practical applications, aimed at improving the lives of individuals with neurological disorders.
It is clear that understanding nerve complexes requires a confluence of expertise and perspectives from various disciplines.
Ending
The exploration of nerve complexes is essential, not only for understanding their immediate structural and functional characteristics but also for recognizing their long-lasting implications for various disciplines. Nerve complexes play a central role in mediating communications within biological systems, facilitating intricate interactions between sensory inputs and motor outputs. Moreover, the continuous advancement in scientific research about these structures yields critical benefits for a plethora of fields, including medicine, psychology, and even artificial intelligence.
One main benefit discussed in this article is the role nerve complexes play in sensory processing. They allow organisms to respond appropriately to changes in their environment, which is crucial for survival. Additionally, understanding how nerve complexes function and interact can lead to improved therapeutic interventions for neurological disorders.
Several considerations arise when reflecting on the conclusion of this article. First, the complexity of nerve structures demands an interdisciplinary approach to fully grasp their significance. Second, the ongoing research in this area holds the promise of revealing further nuances regarding neural communications, which can lead to novel applications in technology and health care.
"Understanding nerve complexes is fundamental for progressing in both scientific inquiry and practical applications in health sciences."
Summary of Key Points
To summarize the essential information presented:
- Structural Integrity: Nerve complexes consist of neurons and glial cells that together form intricate networks facilitating communication.
- Functionality: They are central to processing sensory inputs, coordinating motor responses, and mediating cognitive functions.
- Clinical Relevance: Understanding nerve complexes aids in addressing injuries, neurological disorders, and developing therapeutic interventions.
- Research Advancements: Current studies on neuroplasticity and neural networks are revealing new dimensions of how nerve complexes operate and adapt.
Implications for Future Research
Future research in nerve complexes should focus on several critical areas:
- Interdisciplinary Coordination: Collaboration between neuroscientists, psychologists, and engineers can provide broader insights into nerve complex functions and their applications.
- Neuroplasticity Studies: Continued research in neuroplasticity will enhance our understanding of how these systems adapt, which can lead to better rehabilitation techniques.
- Impact of Technology: Investigating how nerve complexes can interact with developing technologies, like neural interfaces, could lead to breakthroughs in treatment and communication.