Exploring Polymer Synthesis and Its Applications
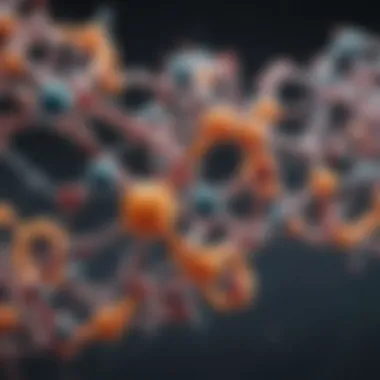
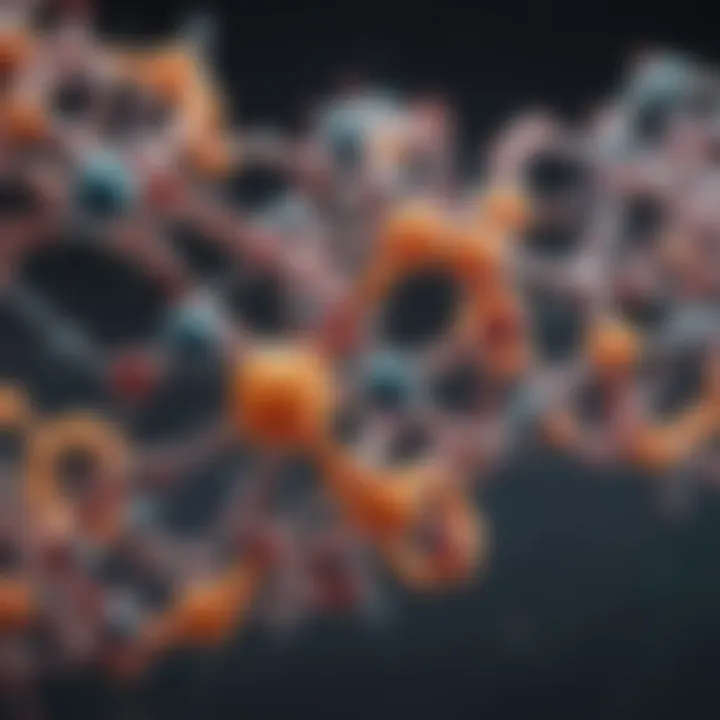
Intro
The creation of polymers involves a blend of complex chemical processes that play a pivotal role in numerous industries. Understanding these processes is not merely an academic pursuit; it is essential for practical advancements in technology, medicine, and material sciences. As polymers form the backbone of many products that meet our daily needs, from packaging materials to high-performance composites, a grasp of their synthesis is crucial.
The significance of polymer synthesis cannot be overstated. It provides the foundational knowledge required to innovate and improve existing materials. Engaging with the methodologies behind polymer creation allows professionals in the field to predict behaviors and tailor properties to specific applications. Examining the intricacies of chemical mechanisms illuminates the path from monomers to polymers, including how their interactions dictate the final characteristics.
This article seeks to unravel the complexities surrounding the synthesis of polymers. It will encompass the essential terminology, diverse methodologies, and significant characteristics that define various polymer types. Special attention will be devoted to the processes of addition and condensation reactions, offering insights into why these methodologies are chosen. Ultimately, by articulating the intricate workings of polymer creation, we aim to enhance the reader's understanding and appreciation of this vital field.
Prologue to Polymers
The study of polymers is essential in comprehending a vast array of materials that significantly impact our lives. From everyday items like plastic bottles to advanced medical devices, polymers play a crucial role in various industries. This section lays the groundwork for understanding their structure, behavior, and usage.
Polymers, which are large molecules composed of repeating units called monomers, are integral to both natural and synthetic materials. Understanding polymers is not just academic; it holds practical importance for innovation and application in fields such as materials science, medicine, and environmental technology. Knowledge of polymers allows researchers and engineers to design better materials and solutions for modern challenges.
Fostering a solid grasp of polymer science leads to numerous benefits. It enhances material selection in product design, promotes advancements in sustainability practices, and informs the creation of polymers that meet specific criteria for performance and safety. This introductory exploration of polymers will provide insights into their definitions, usage, and historical significance.
Definition of a Polymer
A polymer is defined as a large molecule made up of many repeating subunits, known as monomers. These monomers are connected through chemical bonds, forming long chains that define the polymer's physical properties. Polymers can be categorized into two main types: natural and synthetic. Natural polymers occur in nature, such as proteins and cellulose, whereas synthetic polymers are man-made, like nylon and polyethylene.
The unique features of polymers, such as their flexibility, strength, and resistance to temperature changes, stem from their molecular structure. The arrangement of monomers and the type of chemical bonds influence their mechanical and thermal properties.
History of Polymer Science
The history of polymer science dates back centuries, though significant advancements began in the 19th century. Early examples include the discovery of natural rubber by indigenous people. In 1839, Charles Goodyear developed the process of vulcanization, which considerably enhanced the durability of rubber.
The term "polymer" itself emerged in the context of chemistry in the early 20th century. Hermann Staudinger, a German chemist, proposed the concept of macromolecules, challenging previous ideas about how materials form. He was awarded the Nobel Prize in Chemistry in 1953 for his contributions to polymer chemistry.
The subsequent decades witnessed the rise of synthetic polymers invented for various practical applications. For instance, Bakelite, created by Leo Baekeland in 1907, became the first fully synthetic plastic, marking a significant milestone in polymer science.
With ongoing research and development, the field continues to evolve, leading to innovative materials that address current challenges and improve life quality globally. Understanding this historical context enriches the knowledge of present-day polymer creation and application.
Types of Polymers
Understanding the types of polymers is essential because it provides insight into their properties, applications, and behaviors. Polymers can broadly be classified into three categories: natural polymers, synthetic polymers, and biodegradable polymers. Each type serves distinct purposes and has specific characteristics that make them suitable for various applications. This categorization plays a crucial role in determining how polymers behave under different conditions, their environmental impact, and their usability in everyday products.
Natural Polymers
Natural polymers are those found in nature, often derived from living organisms. Examples include cellulose, proteins, and natural rubber. These polymers are formed through biological processes and usually exhibit excellent biocompatibility and biodegradability.
The importance of natural polymers lies in their sustainability and compatibility with the environment. They are often used in sectors like food packaging, textiles, and pharmaceuticals due to their non-toxic nature. Additionally, they usually exhibit remarkable physical properties, making them suitable for diverse applications. Natural polymers can also possess unique structures that, when manipulated, can yield innovative materials for various uses.
In agriculture, for instance, natural polymers like chitosan are valuable for creating biodegradable films. They enhance soil fertility and are safe for plants and animals. However, one must consider the variability in their properties, as they can depend heavily on the source and processing methods, which may affect their effectiveness.
Synthetic Polymers
Synthetic polymers are artificially created through chemical processes. Common examples include polyethylene, polystyrene, and nylon. These materials are designed to mimic or enhance the properties of natural polymers while offering superior performance in specific applications.
The significance of synthetic polymers lies in their wide range of applications across industries. They can be engineered to achieve desired attributes such as strength, flexibility, and resistance to weathering. This tailoring enables their use in sectors ranging from automotive to consumer goods. However, their production raises environmental concerns due to non-biodegradability and the potential for pollution during manufacturing.
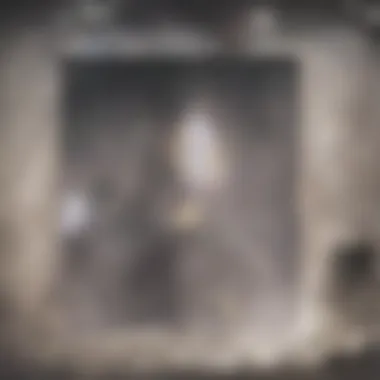
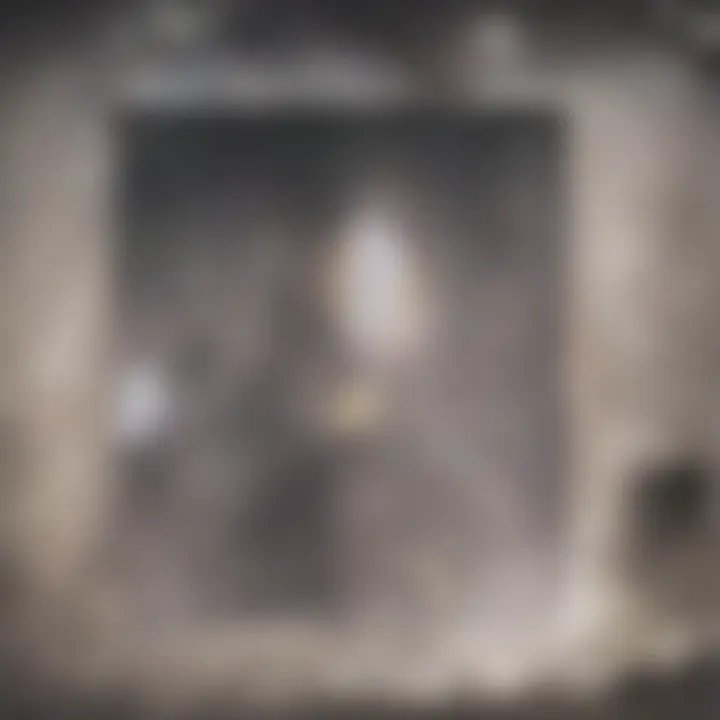
Synthetic polymers can be further categorized based on their properties. For instance, thermoplastics can be reshaped when heated, while thermosets become irreversibly hardened. This diversity allows for versatility in application, but it also calls for a careful examination of life cycle management and recycling efforts.
Biodegradable Polymers
Biodegradable polymers are a subset of synthetic polymers designed to break down under specific environmental conditions. Examples include polylactic acid (PLA) and polyhydroxyalkanoates (PHA). These materials are developed to mitigate the environmental impact of plastic waste.
The relevance of biodegradable polymers is increasingly evident as awareness of plastic pollution grows. They are engineered to decompose naturally through microbial action, thereby reducing the burden of long-lasting plastic waste in landfills and oceans. Their applications range from packaging materials to medical sutures, where breakdown occurs at predictable rates, leaving no harmful residue.
One of the key considerations for biodegradable polymers is their degradation conditions. Factors like temperature, humidity, and microbial activity influence their breakdown rate. Thus, understanding the specific application environment is crucial for their effective use. Furthermore, development in this field aims at improving the mechanical properties of these polymers, making them more suitable for various applications.
Fundamental Principles of Polymer Chemistry
The study of polymer chemistry is fundamental for understanding the very nature of polymers. It deals with the ways these large molecules are formed and how their structural attributes influence their characteristics. Polymer chemistry delves into various reactions and properties that determine how the polymers will behave in different applications. This understanding is crucial for applications in various fields, from materials science to biotechnology. Polymers are ubiquitous in modern life and are associated with diverse functionalities.
Monomers and Polymerization
Monomers are the building blocks of polymers. Alone, these small molecules may have limited utility, but when chemically bonded through polymerization, they give rise to materials with vastly different properties. Polymerization is a chemical process where monomers link together in a repetitive fashion to form a larger structure, known as a polymer.
There are two principal types of polymerization: addition and condensation. Monomers in addition polymerization contain a double bond or some reactive group that can form new bonds with other monomers through the addition of these groups. This leads to an increase in molecular weight without the loss of any small molecules. Condensation polymerization, on the other hand, involves reactions where monomers release a small molecule, like water, during the formation of the polymer.
"Understanding monomers and polymerization is key to manipulating polymer properties for intended applications."
Addition versus Condensation Polymerization
The distinction between addition and condensation polymerization is essential for polymer chemists. In addition polymerization, the process begins with unsaturated monomers, which participate in the reaction without the loss of any atoms. This method typically involves initiators, such as free radicals, which trigger the polymerization. The resulting polymers can range from rubber-like to rigid plastics, depending on the nature of the monomer and reaction conditions. Common examples include polyethylene and polystyrene.
Conversely, condensation polymerization occurs between two different types of monomers, each with a reactive functional group. The elimination of a small molecule is an intrinsic part of this process. This particular type of polymerization can yield polymers such as nylon and polyester, which have distinct characteristics useful for textiles and engineering applications. The selection of polymerization type directly influences the final material properties, such as thermal stability and tensile strength.
Chain Growth and Step Growth
The mechanisms of chain growth and step growth polymerization further elucidate the ways in which polymers are synthesized. Chain growth polymerization is characterized by the rapid addition of monomers to an active site on a growing polymer chain. This process typically has three stages: initiation, propagation, and termination. It tends to produce polymers with high molecular weights and can happen quickly under the right conditions.
Step growth, or step polymerization, on the other hand, involves the reaction of two different kinds of monomers with each having at least two reactive functional groups. In contrast to chain growth, the molecular weight increases gradually in step growth. No pre-existing active centers are required here. The final polymer properties emerge based on the balance between the monomers and reaction conditions
Overall, comprehending these fundamental principles gives researchers insights into how to create polymers that suit specific needs in industry and research. This knowledge not only enhances the understanding of polymer synthesis but also drives innovation in the creation of new materials.
Polymerization Methods
The methods utilized for polymerization are critical in the field of polymer science. They not only influence the properties of the resulting polymers but also determine their suitability for various applications. In this section, we will explore the significant methods of polymerization, discussing the advantages and relevant considerations for each. Understanding these methods can guide researchers and professionals in selecting the appropriate approach for their needs.
Bulk Polymerization
Bulk polymerization involves the formation of polymers from monomers without any solvent or dispersing medium. This method is often preferred due to its simplicity and high productivity. The main components include the monomers, initiator, and temperature. Since no solvent is used, the resulting polymer is typically of high purity, which is advantageous for many applications. However, the exothermic nature of the reaction needs to be managed carefully to prevent overheating.
Some important points about bulk polymerization include:
- Higher Yield: The absence of a solvent contributes to a higher yield of the desired polymer.
- Cost-Effectiveness: The elimination of solvents can reduce production costs.
- Temperature Control: Effective management of heat release is crucial.
Solution Polymerization
Solution polymerization is performed by dissolving the monomers in a suitable solvent. This method is ideal for controlling reaction conditions and properties of the polymer. By adjusting the solvent, temperature, and concentration of monomers, it is possible to influence molecular weight and viscosity of the final product. Furthermore, it allows for the incorporation of additives or fillers.
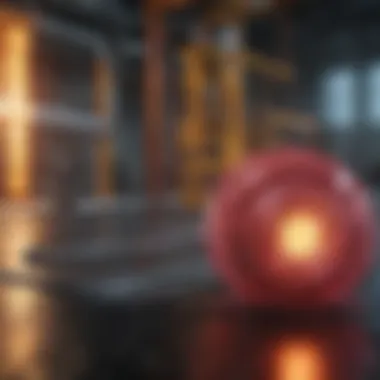
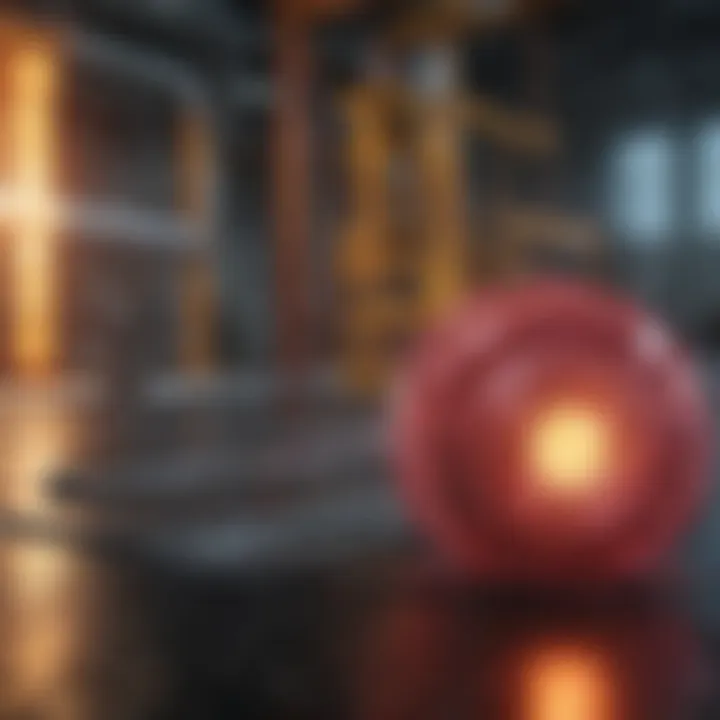
Key aspects of solution polymerization include:
- Controlled Environment: Solvents provide a medium for better control over reaction kinetics.
- Versatile Products: Creates a wide variety of polymer types with specific characteristics.
- Post-Processing: The polymer can be quickly removed from the solution and processed afterward.
Suspension and Emulsion Polymerization
Both suspension and emulsion polymerization are used to create polymers in a stable suspension. They rely on the formation of small droplets of monomers dispersed in water or another continuous phase. Suspension polymerization often yields larger polymer beads, while emulsion polymerization results in smaller particles dispersed in water. Each method has its advantages based on the desired properties of the polymer.
- Suspension Polymerization:
- Emulsion Polymerization:
- Creates larger granules, facilitating easy handling and processing.
- Lower viscosity compared to bulk processes.
- Can handle higher concentrations of solids.
- Faster reaction rates due to a large surface area of monomers.
- Produces fine particles that can be used for coatings and adhesives.
- Reduces the amount of organic solvent required.
Understanding these methods allows scientists to tailor properties for specific applications, ultimately leading to innovative uses of polymers in various fields.
Factors Affecting Polymer Properties
The properties of polymers are integral to their performance in various applications. Understanding these characteristics allows scientists and engineers to tailor polymers for specific uses. This section outlines critical factors that influence the properties of polymers, such as molecular weight, crystallinity, and cross-linking.
Molecular Weight and Distribution
Molecular weight plays a vital role in determining the physical properties of polymers. It directly affects tensile strength, viscosity, and melting point. Polymers with high molecular weights tend to have greater strength and durability but may also be more difficult to process. The distribution of molecular weight within a polymer sample can also influence its behavior. A narrow molecular weight distribution often results in uniform properties, whereas a broad distribution can lead to varied characteristics. Therefore, controlling the molecular weight and understanding its distribution is critical during the synthesis of polymers.
Crystallinity and Amorphous Structure
The arrangement of polymer chains affects the crystallinity and amorphous nature of the material. Crystalline regions provide rigidity, while amorphous regions allow for flexibility. Generally, higher crystallinity results in better tensile strength and thermal stability. Conversely, more amorphous polymers can exhibit lower density and increased impact resistance. The balance between crystallinity and amorphosity is essential when designing polymers for specific applications, such as packaging or structural components.
Cross-Linking and Polymer Networks
Cross-linking refers to the connections formed between polymer chains, influencing the overall network structure. Highly cross-linked polymers tend to have increased mechanical strength, chemical resistance, and thermal stability. On the other hand, flexible polymers may have fewer cross-links, making them more adaptable. The extent of cross-linking can be tailored during the polymerization process, leading to diverse material properties. Thus, understanding how cross-linking affects polymer networks is fundamental for developing advanced materials for various industrial applications.
"The properties of polymers are defined by their molecular weight, crystallinity, and network structure, affecting their performance in diverse applications."
In summary, the interplay between molecular weight, crystallinity, and cross-linking defines a polymer’s overall characteristics. An informed approach when considering these factors can lead to significant advancements in polymer science as well as improving practical implementations.
Applications of Polymers
Polymers play a vital role in modern society, influencing various industries and applications. Understanding their applications assists in appreciating their significance across diverse fields. The integration of polymers into everyday products demonstrates their versatility, performance capabilities, and functional benefits. They contribute to advancements in technology, healthcare, and environmental sustainability. This section outlines key use cases of polymers, each having profound implications on both industry practices and consumer experiences.
Industrial Uses
Polymers are fundamental to many industrial processes. They serve multiple purposes, from packaging materials to components used in machinery. Understanding how polymers function in industrial applications helps to recognize their impact on efficiency and innovation. Some common uses include:
- Packaging Materials: Polymers like polyethylene and polypropylene are widely used in packaging. They provide durability, moisture resistance, and lightweight properties. This helps in reducing transportation costs and extending product shelf life.
- Construction Materials: Plastics contribute significantly to construction through pipes, insulation, and structural elements. Their resistance to corrosion makes them ideal for long-lasting infrastructure.
- Automotive Components: Polymers are present in parts like dashboards, bumpers, and fuel tanks. These materials offer lightweight properties, improving fuel efficiency while maintaining safety.
Medical Applications
In the medical field, polymers are essential for enhancing healthcare solutions. Their application in medical devices and pharmaceuticals showcases innovation. Polymers provide compatibility with biological systems, essential for medical uses. Notable applications include:
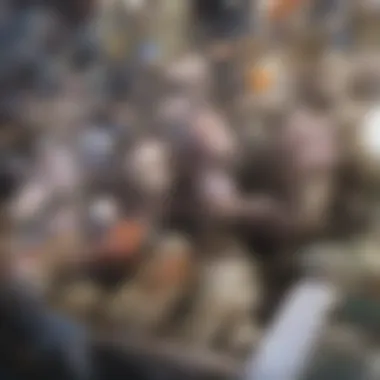
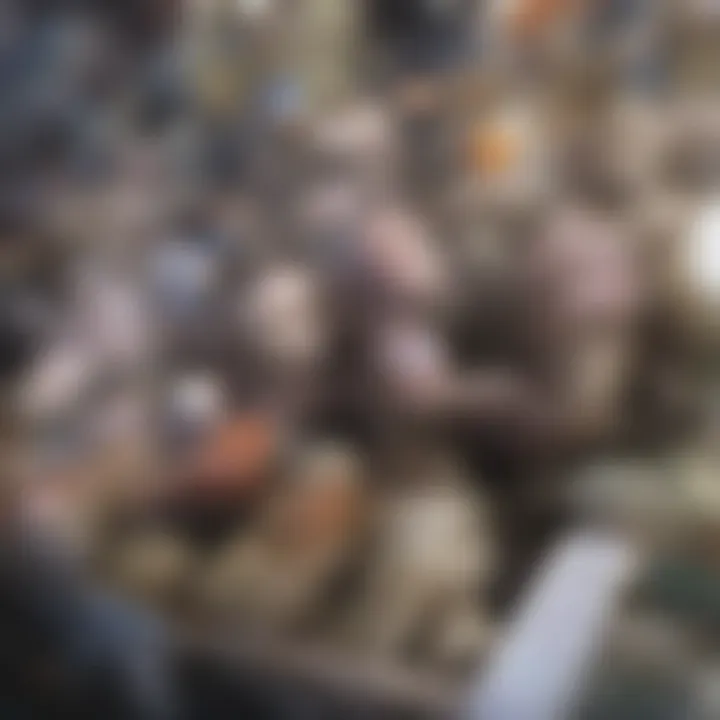
- Biodegradable Implants: Polymers like polylactic acid serve in the development of implants that safely dissolve in the body. This property streamlines recovery and reduces surgical interventions.
- Drug Delivery Systems: Controlled release formulations utilize polymers to target specific areas in the body. Their capability to encapsulate drugs allows for sustained release and improves treatment efficacy.
- Wound Dressings: Polymers create moisture-retentive dressings that promote healing. Hydrogels and polyurethane dressings assist in providing a conducive environment for wound recovery.
Environmental Considerations
As the world faces increasing environmental challenges, the role of polymers in sustainability becomes paramount. Their production, use, and disposal have raised the necessity for environmental consciousness. Considerations in polymer applications include:
- Recycling Programs: Effective recycling of polymers can reduce waste. Many thermoplastics can be reprocessed, keeping materials in circulation and minimizing landfill impact.
- Biodegradable Options: The development of biodegradable polymers, such as polyhydroxyalkanoates, demonstrates efforts to reduce long-term environmental impacts. These materials break down more naturally, decreasing pollution.
- Life Cycle Assessment: Understanding the life cycle of polymers from production to disposal aids in evaluating their environmental footprint. Decisions made in polymer selection can greatly impact sustainability goals.
"Polymers are more than just materials; they are key drivers of innovation across industries. Their applications can contribute both positively and negatively to environmental sustainability."
The applications of polymers span across critical sectors. From industrial uses that enhance productivity to medical applications that improve patient care, their importance cannot be understated. A deep understanding of these aspects allows for more informed choices in both research and practical applications.
Future Directions in Polymer Research
Research in the field of polymers is not static; it continually evolves. Understanding future directions in this discipline is critical for those invested in polymer science, whether they are students, researchers, or industry professionals. These directions can significantly influence the development and application of polymers, leading to breakthroughs in technology, healthcare, and environmental sustainability.
Advancements in Polymer Synthesis
Advancements in polymer synthesis represent a cornerstone of modern polymer research. Methods to create polymers are becoming more sophisticated and efficient. New techniques such as controlled radical polymerization and click chemistry are gaining traction. These methods allow for greater control over polymer architecture and properties.
This level of control can lead to polymers that are precisely tailored for specific applications. Consider the potential of these innovations in creating drug delivery systems that can release medication at controlled rates or smart materials that change properties in response to stimuli.
Innovations in Material Properties
Innovations in material properties are also crucial. Materials scientists are exploring ways to enhance the functionality of polymers. Modifications at the molecular level can lead to enhanced strength, flexibility, or thermal stability. For example, advancements in nanocomposites incorporate nanoparticles into polymers, resulting in materials with superior mechanical and thermal properties.
These enhanced materials can find use in a myriad of applications, ranging from aerospace components to everyday consumer goods. As researchers continue to investigate how the molecular structure of polymers impacts their properties, the potential for developing high-performance materials increases.
Sustainability Practices in Polymer Production
Sustainability is becoming a focal point in polymer research. As environmental concerns rise, the need for sustainable practices in polymer production is more pressing than ever. Researchers are investigating bio-based feedstocks and recycling methods. This approach could significantly reduce the reliance on fossil fuels and minimize environmental impact.
Sustainable practices also involve the development of biodegradable polymers. These materials can reduce long-term waste in landfills and oceans, thus addressing a significant environmental challenge. As these innovations mature, we will likely see a shift in how the industry approaches polymer production.
"Future directions in polymer research not only focus on innovation but also address ethical and environmental challenges, making it a crucial field for sustainable development."
The future of polymer research holds immense promise. Innovations in synthesis, material properties, and sustainability practices have far-reaching implications across various sectors. By actively engaging with these developments, the scientific community can help shape a more sustainable and technologically advanced world.
Closure
In recapitulating key points, we are reminded of the varied types of polymers, the fundamental principles underpinning their synthesis, and the diverse methods employed in their production. These takeaways underscore the remarkable versatility and applicability of polymers in everyday life, as well as their impact on technological advancement.
Moreover, the importance of specific characteristics—such as molecular weight, crystallinity, and cross-linking—is highlighted, emphasizing how these properties influence a polymer’s performance in real-world applications. These considerations are vital for anyone engaged in polymer research or industry, as they directly affect the development of new materials and technologies.
Let us also consider the benefits that arise from understanding polymer synthesis. Advancements in material properties can lead to breakthroughs in fields like energy storage, environmental sustainability, and healthcare solutions. Thus, recognizing the significance of polymer science in these contexts prompts a more profound appreciation for this discipline.
Recapitulation of Key Points
- Polymer Diversity: The article discusses various types of polymers, such as natural, synthetic, and biodegradable polymers, each serving distinct functions in the modern landscape.
- Synthesis Methods: Different polymerization methods, including bulk and solution techniques, are explored, shedding light on their unique characteristics and processing challenges.
- Influence of Properties: Key properties affecting polymer performance, like molecular weight and crystalline structure, are detailed, providing insight into their practical applications.
Implications for Future Studies
As we reflect on the insights from this article, it becomes evident that future studies in polymer science hold vast potential. Several directions can be identified:
- Innovative Synthesis Techniques: There is a continuous demand for novel methods in polymer synthesis that can improve efficiency and reduce environmental impacts.
- Material Optimization: Further research could lead to the development of polymers with tailored properties, enhancing their applications in cutting-edge technologies such as nanotechnology and medical devices.
- Sustainability Initiatives: Researching biodegradable polymers and sustainable practices in polymer production is increasingly important, particularly as environmental concerns continue to grow.
By navigating these areas, researchers and practitioners can contribute to the advancement of polymer science, ultimately leading to innovations that benefit society as a whole.