Hydrogen to Electricity: Mechanisms and Future Prospects
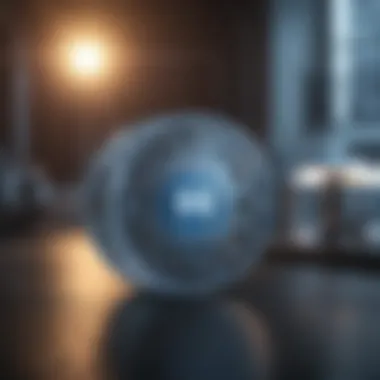
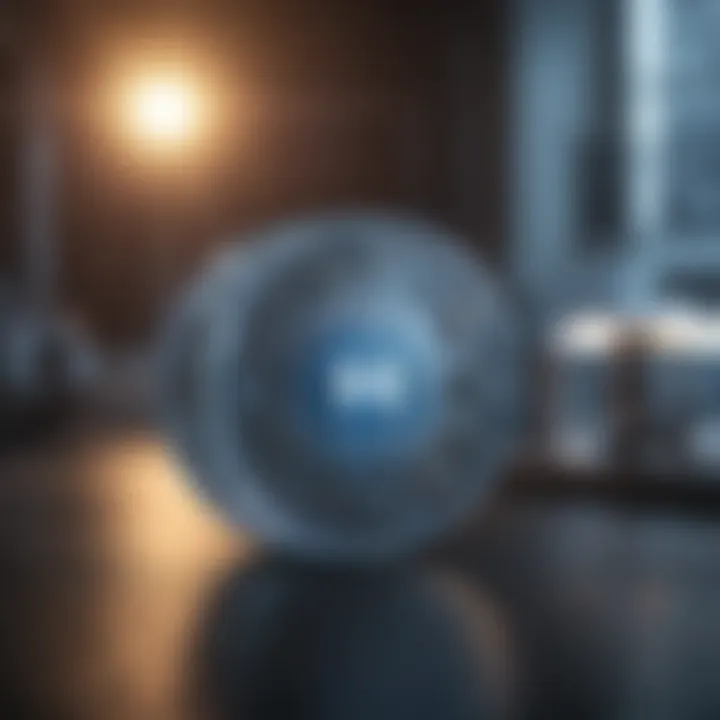
Intro
Hydrogen is gaining significant attention as a potential alternative energy source. Its role in sustainable energy is being reexamined as global efforts shift towards reducing dependence on fossil fuels. This shift brings forth several questions regarding the mechanisms involved in converting hydrogen to electricity. Understanding the core concepts, the technologies available, and their implications becomes essential for various stakeholders including students, researchers, educators, and professionals.
This article will delve into crucial aspects surrounding the transformation of hydrogen into electricity. The process of hydrogen production, fuel cell technology, and future energy systems using hydrogen will be explored. We will also assess the potential environmental impacts and economic factors associated with this transition. Through this overview, we aim to provide a comprehensive understanding of hydrogen's importance in the energy landscape.
Preface to Hydrogen as an Energy Carrier
Hydrogen has emerged as a pivotal energy carrier in the pursuit of sustainable energy solutions. Its ability to efficiently store and release energy positions it as a favorable alternative to traditional energy sources. The significance of hydrogen lies not just in its potential for fueling various applications, but also in its role in facilitating the transition towards a low-carbon economy. Hydrogen, when converted to electricity, holds the promise of reducing our environmental footprint, aligning with ambitious climate goals and energy demands of the future.
A key element of hydrogen's importance as an energy carrier is its versatility. It can be utilized across multiple sectors, including transportation, industrial processes, and residential energy systems. Additionally, hydrogen can be produced from numerous resources, be it renewable sources or fossil fuels, making it adaptable for a wide range of applications.
Definition of Energy Carriers
Energy carriers are mediums that store, transport, and deliver energy across different systems. They can take various forms including solid, liquid, or gas. Energy carriers are essential for moving energy from production to consumption points, ensuring that it can serve various functions when needed. Hydrogen represents a unique energy carrier because it can be produced from diverse sources, stored in different states, and utilized in multiple applications. Its efficiency in transferring energy highlights its growing relevance in modern energy systems.
Hydrogen's Unique Properties
Hydrogen possesses several distinct properties that make it an advantageous energy carrier. First, it is the lightest and most abundant element in the universe, enabling easy storage and transportation. Second, hydrogen exhibits a high energy content per unit mass, which means it can release substantial energy when converted into electricity. Third, when hydrogen undergoes combustion or is used in fuel cells, the primary byproduct is water. This characteristic significantly minimizes environmental impact, as opposed to traditional fossil fuels that release harmful emissions.
In summary, hydrogen’s multifaceted attributes not only reinforce its position as an energy carrier but also pave the way for innovative applications. As we delve deeper into its conversion processes and implications, understanding these foundational aspects is crucial to appreciate the broader scope of hydrogen within energy systems.
Historical Context of Hydrogen Use
Understanding the historical context of hydrogen use is important in grasping its current role in our energy systems. The journey of hydrogen, from early applications to modern technologies, illustrates not only the potential of this element but also the challenges faced in harnessing its energy. This historical backdrop provides insights into the evolution of hydrogen technologies and the lessons learned throughout this process.
Early Applications of Hydrogen
Hydrogen was first discovered in the late 18th century by Henry Cavendish, who identified it as a distinct element. Early applications of hydrogen centered around its unique properties. Initially, it was used in balloons for flight. The Hindenburg disaster in 1937 highlighted the dangers associated with hydrogen, leading to a shift towards helium. Despite this setback, hydrogen's potential as a fuel source did not go unnoticed.
In the 19th century, hydrogen began to gain attention for its possible applications in energy. The ability to produce energy through combustion was explored, setting the stage for later developments. Notably, chemist William Grove created the first fuel cell in 1839, which combined hydrogen and oxygen to generate electricity, laying foundational concepts for hydrogen electricity.
Evolution of Hydrogen Technologies
The evolution of hydrogen technologies spans many decades and reflects advancements in understanding and engineering. During the 20th century, research intensified, particularly during WW II and the space race, when hydrogen played a crucial role as a rocket propellant. The Apollo program utilized liquid hydrogen for its Saturn V rockets, establishing hydrogen as a viable energy source in aerospace applications.
In the latter part of the century, environmental concerns surged. The 1970s oil crisis caused nations to search for alternative energy sources, prompting renewed interest in hydrogen. Researchers began examining methods of hydrogen production, including steam methane reforming and electrolysis, which emphasized generating hydrogen sustainably.
By the early 21st century, energy policy shifted towards renewable energy, and hydrogen technology continued to mature. Emerging fuel cell technologies, including Polymer Electrolyte Membrane and Solid Oxide Fuel Cells, demonstrated efficiency improvements and potential for broader applications.
As the world addresses climate change, the historical context of hydrogen use is more relevant than ever. Today's discussion around hydrogen emphasizes its role in a sustainable energy future. Understanding this journey helps frame current advancements, considerations, and the implications of integrating hydrogen into our energy systems.
“The evolution of hydrogen use illustrates both its challenges and its promise as a key player in sustainable energy systems of the future.”
In summary, appreciating the historical context of hydrogen use provides clarity in recognizing its potential and challenges. As technology progresses, so do our perspectives on hydrogen's feasibility, making it an essential focus in the transition to cleaner energy.
While hydrogen has been tried and tested over the years, ongoing innovations continue to redefine its applications, informing a bright future for hydrogen in the global energy landscape.
Methods of Hydrogen Production
Hydrogen production represents a key aspect in the energy transition, emphasizing its role as an essential energy carrier. The methods to produce hydrogen are varied and each method has its own benefits and drawbacks. Understanding these methodologies can aid in assessing their applicability in a sustainable energy landscape. This section explores the principal hydrogen production methods, focusing on their operations and implications.
Steam Methane Reforming
Steam methane reforming (SMR) is one of the most widely used methods for hydrogen production. In this process, methane from natural gas reacts with steam in the presence of a catalyst to produce hydrogen and carbon monoxide. The reaction occurs at high temperatures, typically between 700 to 1000 degrees Celsius. SMR's efficiency is notable, producing hydrogen at a relatively low cost; however, it still relies heavily on fossil fuels.
Factors to consider include:
- Carbon Emissions: Utilizing natural gas leads to CO2 emissions during the reaction.
- Economic Viability: The infrastructure for natural gas is well-established, which lowers operational costs.
- Scalability: Facilities can be built to accommodate large output needs, making it suitable for industrial applications.
Electrolysis of Water
Electrolysis is a promising method for hydrogen production that separates water into hydrogen and oxygen using electricity. The process involves passing an electric current through water containing an electrolyte, which facilitates the reaction. This method is particularly valuable when renewable energy sources provide the electricity, leading to a low-carbon hydrogen product.
Key points about electrolysis include:
- Renewable Integration: Hydrogen produced this way is often termed "green hydrogen", due to its connection with renewable energy.
- Energy Source Variability: The efficiency of electrolysis depends on the source of electricity used, impacting overall cost and environmental benefit.
- Technological Advancements: Improvements in electrolysis technology, such as proton exchange membrane electrolysis, are refining efficiency.
Biological Hydrogen Production
Biological hydrogen production leverages microorganisms to produce hydrogen gas. Certain bacteria, algae, and even enzymes can convert organic matter into hydrogen. This process often occurs through fermentation or photosynthesis and shows great potential for sustainable hydrogen generation.
Considerations for biological production include:
- Feedstock Diversity: Various organic materials can be used, which makes this method flexible and adaptable.
- Low Energy Input: The biological processes require less energy input compared to traditional methods.
- System Complexity: Biological systems can be less predictable and control mechanisms can be challenging.
Hydrogen from Biomass
Producing hydrogen from biomass involves converting organic materials into hydrogen through thermal, chemical, or biological processes. Gasification and pyrolysis are two typical methods in this category. This approach not only generates hydrogen but also offers a way to utilize waste materials, aiding waste management.
Important aspects include:
- Waste to Energy: Utilizing biomass contributes to resource management while generating energy.
- Carbon Neutrality: Many biomass sources are considered carbon neutral, contributing to sustainability goals.
- Market Availability: The availability of raw materials may vary, affecting output and consistency.
Hydrogen Storage and Distribution
The storage and distribution of hydrogen are integral aspects of its role as an energy carrier. Efficient storage solutions ensure that hydrogen can be transported and utilized when and where it is needed. The conversation around hydrogen typically centers on its production and conversion into electricity, but without a robust infrastructure for storage and distribution, these processes cannot be fully realized. This section will explore some prominent methods of hydrogen storage, each with unique benefits and considerations, to highlight their importance in the overall system of hydrogen energy.
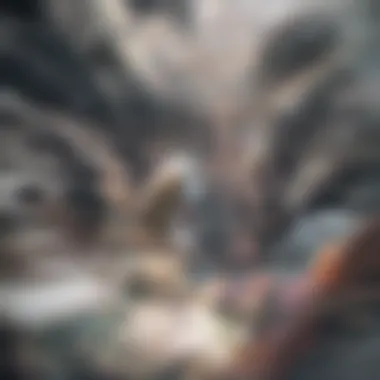
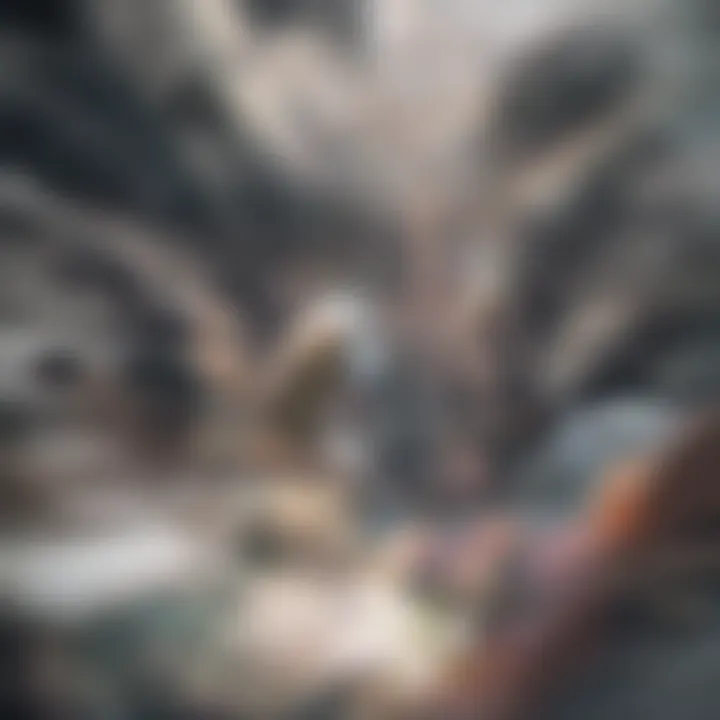
Compressed Hydrogen Storage
Compressed hydrogen storage involves storing hydrogen gas at high pressures typically ranging from 350 to 700 bar. This method is widely used due to its relatively simple technology and established experience in the field. Despite being efficient, several factors influence its popularity and effectiveness.
- Advantages:
- Considerations:
- Space-efficient: Compressed hydrogen has a high energy density, allowing storage in smaller volumes compared to other methods.
- Availability: This technology is already in use for several applications, including hydrogen fuel cell vehicles.
- Safety: High-pressure storage introduces risks of leaks and bursts, necessitating rigorous safety protocols.
- Cost: The price of tanks and infrastructure can be high, posing a challenge for widespread adoption.
Liquid Hydrogen Storage
Liquid hydrogen storage involves cooling hydrogen gas to extremely low temperatures (around -253°C) to convert it into a liquid form. This method is another viable option for long-term storage due to its higher volumetric energy density compared to gaseous storage.
- Advantages:
- Considerations:
- Higher Density: Liquid hydrogen offers a much denser form of storage, which is advantageous for transport over long distances.
- Efficiency: This method is suitable for applications requiring large hydrogen volumes, such as in space exploration.
- Complex Technology: The systems required to liquefy and maintain hydrogen at low temperatures are complex and costly.
- Evaporation Loss: Even with good insulation, some loss of hydrogen occurs through evaporation, which can affect the overall efficiency.
Metal Hydrides
Metal hydrides are stable compounds formed by hydrogen and metals, enabling hydrogen storage in a solid-state form. This method represents a potential solution to some challenges associated with gaseous and liquid hydrogen storage.
- Advantages:
- Considerations:
- Safety: Metal hydrides operate at lower pressures and temperatures than gaseous methods, reducing risks associated with high-pressure storage.
- Compactness: This form of storage can be more compact, making it suitable for applications such as portable power devices.
- Release Rate: The hydrogen release rate from metal hydrides can be slower than desired for some applications, impacting responsiveness.
- Material Costs: The materials used in metal hydrides can be expensive, which may limit their economic viability for large-scale solutions.
Hydrogen storage and distribution methods play a pivotal role in harnessing hydrogen's potential as a clean energy source. Understanding the pros and cons of each method helps guide future innovations and investments in hydrogen technology. Safe, efficient, and economical storage solutions will pave the way for broader acceptance and utilization of hydrogen as part of the global energy landscape.
Converting Hydrogen into Electricity
The conversion of hydrogen into electricity is a pivotal aspect of energy transformation. It harnesses hydrogen's unique properties to produce clean energy with low environmental impact. This section explores the mechanisms, advantages, and implications of such conversion while focusing on various technologies and methodologies relevant to this process.
Overview of Fuel Cells
Fuel cells serve as the principal technology for converting hydrogen into electricity. They operate on the basic principle of electrochemical reactions, which combine hydrogen and oxygen to generate electricity, water, and heat. This process occurs continuously as long as the necessary reactants are supplied. Fuel cells offer significant benefits, such as high efficiency and low emissions compared to traditional combustion processes. The usage of fuel cells is thus a crucial element in the context of sustainable energy systems.
Types of Fuel Cells
Understanding the various types of fuel cells is essential for appreciating their applications in converting hydrogen into electricity.
Polymer Electrolyte Membrane Fuel Cells
Polymer Electrolyte Membrane Fuel Cells (PEMFCs) are particularly recognized for their efficiency and rapid start-up capabilities. They operate at low temperatures, making them suitable for various applications, including transportation. A key characteristic of PEMFCs is their ability to achieve high power density. This advantage makes them a popular choice in vehicles like the Toyota Mirai. However, they require pure hydrogen and are sensitive to water management, which can be a drawback in certain scenarios.
Phosphoric Acid Fuel Cells
Phosphoric Acid Fuel Cells (PAFCs) are known for their robustness and durability, often utilized in stationary applications. They operate at higher temperatures than PEMFCs and can handle impure hydrogen, which is a clear advantage. This feature allows PAFCs to be less affected by fuel quality. However, their lower power density and higher operational temperature may limit their attractiveness for mobile applications compared to PEMFCs.
Solid Oxide Fuel Cells
Solid Oxide Fuel Cells (SOFCs) represent another category that operates at much higher temperatures. This high operating temperature allows SOFCs to achieve very high efficiency, particularly when converting hydrocarbon fuels into electricity. Their ability to use a variety of fuels, including natural gas, showcases a unique flexibility in applications. On the downside, the high thermal requirements may lead to longer start-up times and potential materials challenges due to thermal stresses.
Operating Principles of Fuel Cells
Fuel cells convert chemical energy into electrical energy through distinct electrochemical reactions. The basic operating principle involves the anode where hydrogen is supplied, undergoing oxidation to release electrons and protons. The electrons travel through an external circuit, creating an electric current, while protons move through the electrolyte to the cathode. At the cathode, oxygen combines with protons to form water, the only byproduct of this process. This emphasizes the clean and efficient nature of fuel cells as a source of electricity, making them a critical component in future energy systems.
"Fuel cells represent a clean alternative to conventional energy systems, leveraging hydrogen's capabilities to minimize environmental impact."
Efficiency of Hydrogen-to-Electricity Conversion
The efficiency of converting hydrogen into electricity is a central theme in understanding hydrogen as an energy carrier. Efficient conversion is crucial for the practical realization of hydrogen technologies in various applications. High efficiency ensures that more energy is extracted from hydrogen, minimizing waste and optimizing overall system performance. As the world seeks cleaner energy solutions, hydrogen's efficiency becomes vital in reducing greenhouse gas emissions and reliance on fossil fuels.
Factors Influencing Efficiency
Several elements determine how efficiently hydrogen can be converted into electricity. One of the primary factors is the type of fuel cell used. Each fuel cell technology has varying efficiency levels based on its electrochemical processes and operational conditions. Here are specific elements to consider:
- Type of Fuel Cell: For instance, polymer electrolyte membrane fuel cells typically show higher efficiency than phosphoric acid fuel cells in certain applications.
- Operating Temperature: Fuel cells operating at higher temperatures, such as solid oxide fuel cells, can achieve higher efficiencies, due to improved thermodynamic cycles.
- Purity of Hydrogen: The presence of impurities can significantly hinder fuel cell performance, reducing efficiency. Higher purity levels result in better performance and longevity of system components.
- Load Conditions: The efficiency of hydrogen conversion can vary according to load conditions, especially in load-following applications where demand fluctuates.
Improving these factors can lead to enhanced efficiency. Thus, ongoing research focuses on optimizing fuel cell designs and operational parameters to maximize energy output.
Comparative Efficiency with Other Energy Sources
In the context of the broader energy landscape, it is essential to compare hydrogen's efficiency with that of other sources. Hydrogen has unique advantages, despite not being the most efficient in every situation:
- Hydrogen Fuel Cells can achieve efficiencies of around 60%, possibly higher when waste heat is recovered in combined heat and power systems.
- In contrast, internal combustion engines typically have efficiencies between 20% to 30%. This difference highlights hydrogen's potential as a clean energy source.
- Additionally, traditional electricity generation methods, such as coal or natural gas turbines, also have comparable efficiencies but produce substantial carbon emissions.
An important takeaway here is the potential for hydrogen to serve as a bridge in the transition from conventional energy sources to more sustainable alternatives. With advancements in technology, the competitiveness of hydrogen, in terms of efficiency and emissions, will continue to evolve.
"The efficiency of hydrogen-to-electricity conversion is not merely a technical concern; it is pivotal to the acceptance and integration of hydrogen energy systems in the global energy transition."
Applications of Hydrogen Electricity
The applications of hydrogen electricity span a wide range of sectors, demonstrating its versatility and potential as a sustainable energy source. This section delves into how hydrogen is transformed into electricity and the implications of its adoption across various fields. Each application not only showcases technological advancements but also emphasizes the benefits and considerations surrounding the use of hydrogen as an energy carrier.
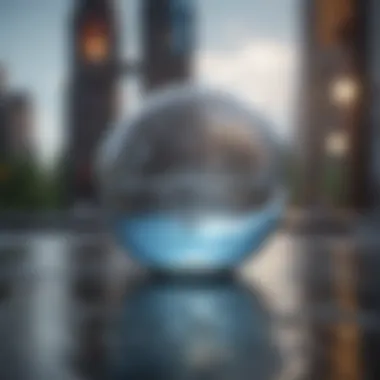
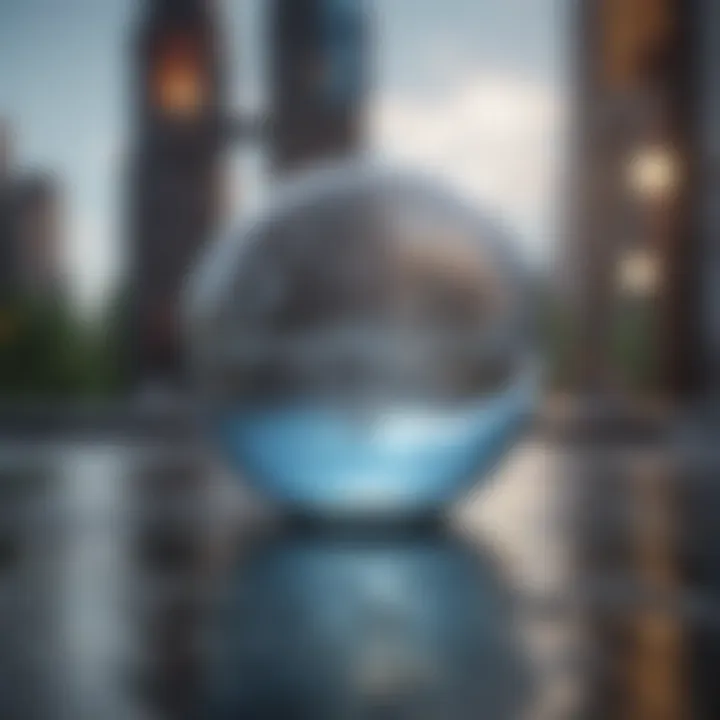
Transportation
Hydrogen's role in transportation is significant as the world seeks cleaner alternatives to fossil fuels. Hydrogen fuel cell vehicles (FCVs) have emerged as a promising solution, converting hydrogen into electricity to power electric motors. This technology offers several advantages. Notably, water vapor is the only byproduct, thus minimizing emissions and improving air quality.
The use of hydrogen in public transport, particularly buses and trains, can reduce urban pollution levels. Moreover, FCVs typically refuel in a matter of minutes compared to the longer charging times of battery-electric vehicles. They also have greater driving ranges, making them suitable for long-distance travel. However, challenges exist. Infrastructure for hydrogen refueling is still limited, impacting widespread adoption.
Industrial Use
In various industries, hydrogen electricity serves as a critical component. The industrial sector often requires high-temperature heat, traditionally supplied by fossil fuels. Hydrogen can replace these fuels, particularly in processes such as steelmaking and chemical production. The benefits here include reducing carbon emissions significantly. For example, using hydrogen as a reducing agent can transform iron ore into iron without emitting carbon dioxide.
Moreover, industries are exploring hydrogen's role in energy storage solutions. When integrated into production systems, hydrogen can act as a buffer, storing excess energy generated from renewable sources. This ensures a more continuous energy supply while promoting greener practices. Nonetheless, the implementation of hydrogen in industry necessitates modifications to existing equipment and infrastructure to accommodate its unique properties.
Power Generation
Hydrogen electricity also finds applications in power generation, contributing to a sustainable energy mix. The burning of hydrogen in power plants or its use in fuel cells provides a clean method for generating electricity. These systems can be utilized in combination with renewable energy sources like wind and solar, helping to balance supply and demand.
Fuel cells can be deployed across grid systems for distributed generation. This approach allows for localized electricity production, potentially reducing reliance on large, centralized power plants. Additionally, hydrogen can serve as an energy carrier, storing surplus energy generated during peak production times for use when demand is higher. Challenges remain in terms of efficiency and cost. As infrastructure develops and economies of scale improve, hydrogen's role in power generation is expected to expand.
"Hydrogen has the potential to reshape energy landscapes across sectors, from transportation to industrial applications and power generation."
The exploration of hydrogen in these application areas navigates both opportunities and obstacles. As research and investments continue, a deeper understanding of implementing hydrogen technologies will emerge, fostering a transition toward a more sustainable energy future.
Environmental Impact of Hydrogen Energy Systems
The exploration of hydrogen energy systems encompasses not only the technical and economic facets but also the crucial environmental impact. Understanding how hydrogen interacts with environmental systems is essential for assessing its viability as a sustainable energy source. This section intends to outline the significance of evaluating hydrogen's environmental footprint, concentrating on its production processes and their ecological implications.
Hydrogen is inherently clean when utilized in fuel cells, as its only by-product is water vapor. However, the path to this clean energy involves methods of production that can impose a substantial carbon footprint. Therefore, examining the carbon footprint of hydrogen production becomes vital in determining whether hydrogen energy systems contribute positively to climate change mitigation.
Carbon Footprint of Hydrogen Production
The methods used to generate hydrogen significantly influence its overall carbon footprint. Some methods, like steam methane reforming (SMR), are widely employed but are also heavy on greenhouse gas emissions. SMR converts natural gas into hydrogen through a process that releases carbon dioxide into the atmosphere. As a result, this approach raises concerns over hydrogen's sustainability, especially compared to renewable methods.
In contrast, methods emphasizing renewable resources, such as electrolysis, can considerably reduce the carbon emissions associated with hydrogen production. If the electricity used in electrolysis comes from wind, solar, or hydroelectric sources, the total greenhouse gas emissions can be drastically minimized. Here are some impacts:
- SMR: Emits approximately **9–12 kg of CO2/kg of ** produced.
- Electrolysis with renewable energy: Can achieve emissions as low as **0.2 kg of CO2/kg of **.
Thus, while hydrogen holds promise for renewable energy transitions, its carbon footprint varies extensively based on production methods. Careful selection and innovative technology adoption are crucial for mitigating negative environmental effects.
Potential Benefits for Climate Change Mitigation
Integrating hydrogen energy systems into the global energy landscape offers various potential benefits for mitigating climate change. Hydrogen has the capacity to store energy generated by intermittent renewable sources, enabling a more stable and reliable energy supply. This characteristic is invaluable in a future where energy systems rely heavily on wind and solar power, which experience fluctuations in availability.
- Energy Storage: Hydrogen enables excess renewable energy to be stored and used during high-demand periods.
- Decarbonization: Transitioning to hydrogen fuel for transportation and industrial processes could significantly lower carbon emissions, especially in sectors difficult to electrify.
- Reducing Air Pollutants: Hydrogen combustion produces only water vapor, unlike fossil fuels that release nitrogen oxides and particulate matter.
Overall, harnessing hydrogen energy systems presents a strategic opportunity in the fight against climate change. Evaluating both its carbon footprint and its multifaceted benefits provides a framework to envision hydrogen as a pivotal component of sustainable energy solutions moving forward.
"Hydrogen energy systems have the potential not only to reduce emissions but also to catalyze a broader transformation towards a sustainable energy future."
Economic Considerations of Hydrogen Energy
The economic aspects of hydrogen energy are crucial for understanding its viability and potential impact on future energy systems. As hydrogen emerges as a sustainable energy source, considerations around its production costs and market dynamics become increasingly significant. This section delves into the economic landscape surrounding hydrogen energy, focusing on production costs and market behaviors that influence pricing and adoption.
Cost of Hydrogen Production
The cost of producing hydrogen varies significantly depending on the method used. The primary production techniques include steam methane reforming, electrolysis, and biological processes. Each method has its own cost structure influenced by factors such as resource availability, technology maturity, and energy demand.
- Steam Methane Reforming (SMR): Currently, SMR is the most widely used method, primarily due to its cost-effectiveness. However, it relies on natural gas, which raises concerns over fossil fuel dependency and associated carbon emissions.
- Electrolysis: This method involves splitting water into hydrogen and oxygen using electricity. The development of renewable energy sources for powering electrolysis could greatly reduce costs and enhance sustainability. However, the high initial investment for electrolyzers and the variable cost of electricity can limit widespread adoption.
- Biological and Biomass Production: These methods are gaining interest. They may have a lower environmental footprint but typically involve longer production times and potentially higher costs on a per-kilogram basis.
In considering the economic feasibility of hydrogen energy, governmental policies and technological innovations play a vital role in driving down production costs. Technological advancements and economies of scale in production techniques can significantly impact overall hydrogen pricing, making it more competitive against traditional fossil fuels.
Market Dynamics and Future Pricing
The market dynamics for hydrogen are influenced by various factors including supply and demand, competing energy sources, and regulatory frameworks. Demand for hydrogen is anticipated to rise due to its applications in transportation, industrial processes, and energy storage.
"As societies seek low-carbon solutions, hydrogen is poised to play a pivotal role in the future energy landscape."
Factors that affect hydrogen pricing can be categorized as follows:
- Supply Chain Development: The infrastructure for hydrogen production, storage, and transportation is still developing in many regions. A robust supply chain can reduce costs and improve reliability, attracting more investment into the market.
- Competing Energy Sources: As renewable technologies like solar and wind continue to expand, they affect the cost structures of hydrogen production, particularly electrolysis.
- Regulatory Support: Government policies that promote hydrogen initiatives or incentivize investments can significantly alter market dynamics. Incentives for cleaner energy sources can drive up hydrogen demand, consequently influencing its pricing.
- Global Market Trends: Hydrogen markets are increasingly interconnected, with international collaborations and trade agreements shaping pricing models. Global developments in hydrogen economies, particularly in Europe and Asia, could ripple through local markets, influencing overall costs.
In summary, the economic considerations of hydrogen energy encompass the intricacies of production costs and market behaviors that are essential for its adoption as a mainstream energy source. As technology advances and global energy paradigms shift towards sustainability, the economic viability of hydrogen will have a profound impact on future energy solutions.
Regulatory and Policy Frameworks
The governance surrounding hydrogen energy is a pivotal aspect influencing its adoption and integration into the energy market. Regulatory and policy frameworks establish the standards and incentives necessary for the development of hydrogen technologies. The importance of this topic lies in its capacity to shape public and private sector engagement with hydrogen solutions, thus promoting sustainable energy practices.
Effective regulatory frameworks provide clarity and assurance to investors and stakeholders. They define safety standards, environmental protocols, and operational guidelines for hydrogen production, storage, and utilization. Such regulations are critical not only to protect public interests but also to facilitate innovation in the hydrogen sector.
In addition, policy frameworks can drive funding toward research and development, making it more attractive for companies to invest in hydrogen solutions. These initiatives can encourage widespread implementation of hydrogen technologies, ultimately reducing reliance on fossil fuels and enhancing energy security.
Furthermore, collaboration between governmental bodies, industries, and academic institutions can foster a favorable climate for hydrogen energy advancements. A well-structured policy environment assures all parties that hydrogen initiatives will be supported, leading to a more robust hydrogen economy.
Current Regulations on Hydrogen Use
Current regulations on hydrogen use vary by country, reflecting different levels of maturity in the hydrogen market. In many jurisdictions, safety and environmental regulations are paramount. For instance, regulations may cover the permissible concentrations of hydrogen in various applications, transport requirements for hydrogen, and standards for hydrogen production facilities.
Often, governments implement safety regulations that align with existing standards set for other gases. Agencies such as the Occupational Safety and Health Administration (OSHA) in the United States oversee compliance in workplaces that involve hydrogen. Similarly, the Department of Transportation regulates the transport of hydrogen to ensure it meets safety criteria.
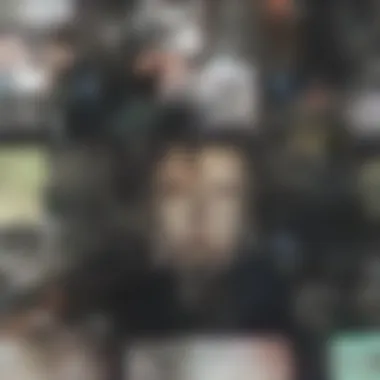
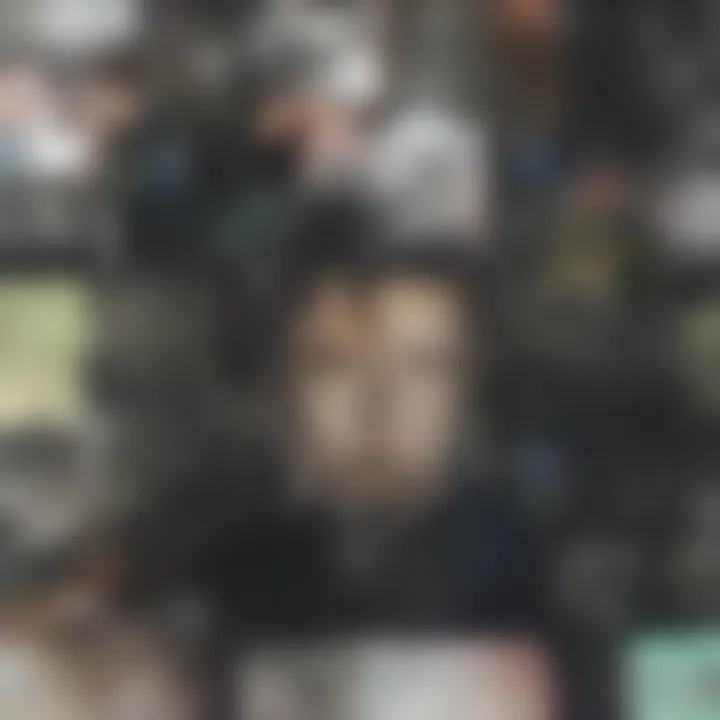
On a broader level, international organizations like the International Organization for Standardization (ISO) have developed standards that affect how hydrogen is produced, stored, and used globally. Countries aiming to establish hydrogen infrastructure must align with these standards while adapting them to their specific contexts.
Incentives for Hydrogen Technologies
Incentives are crucial for stimulating the growth of hydrogen technologies and ensuring they can compete in the energy market. These incentives can take various forms, including financial subsidies, grants for research, tax breaks for companies investing in hydrogen infrastructure, and regulatory support.
Governments worldwide are increasingly recognizing the potential of hydrogen to drive down emissions and provide energy resilience. Therefore, initiatives such as the Hydrogen Production Tax Credit can significantly lower the financial burden on hydrogen producers. This support creates an environment where innovation can thrive and drives better market conditions for hydrogen solutions.
Moreover, public-private partnerships can enable the sharing of resources and knowledge, further accelerating technology advancement. Incentives can also address initial high capital costs associated with hydrogen production and distribution, allowing more players to enter the market.
Future Prospects for Hydrogen Energy Systems
The future of hydrogen energy systems presents a myriad of opportunities and challenges that could reshape our energy landscape. As nations grapple with climate change and energy security, hydrogen emerges as a pivotal player in establishing sustainable energy solutions. The discussion on future prospects must not only highlight advancements in technology but also consider economic viability, regulatory frameworks, and societal acceptance.
One of the key elements in this future is the potential for hydrogen to integrate seamlessly within existing energy structures. For instance, the blending of hydrogen into natural gas pipelines can initiate a transition towards greener energy without major infrastructure disruptions. This transitional strategy could ease the adoption of hydrogen technologies while addressing current energy demands. There is also a growing focus on the development of hydrogen infrastructure, which includes production facilities, storage systems, and distribution networks to ensure hydrogen's accessibility.
Technological Innovations on the Horizon
Several technological innovations are poised to impact hydrogen energy systems significantly. Advanced electrolysis methods, such as proton exchange membrane (PEM) electrolysis, show promise for improving hydrogen production efficiency while minimizing costs. Furthermore, researchers are exploring innovative materials and designs for fuel cells that enhance performance and longevity. Such improvements could facilitate broader adoption in sectors such as transportation and grid storage.
In addition, artificial intelligence and machine learning technologies are being integrated to optimize hydrogen production, storage, and distribution. By predictive analytics, it becomes feasible to manage hydrogen supply chains better, thereby reducing inefficiencies. Overall, these innovations are not merely incremental; they represent a fundamental rethinking of how hydrogen can be produced and utilized effectively.
Hydrogen in a Decarbonized Economy
As the global push toward a decarbonized economy intensifies, hydrogen's role cannot be overstated. Hydrogen has the potential to decouple economic growth from carbon emissions. This can be achieved, particularly when green hydrogen—produced using renewable electricity—is leveraged in high-emission sectors such as steel manufacturing and heavy transportation. The displacement of fossil fuels by hydrogen in these sectors could catalyze monumental reductions in greenhouse gases.
Furthermore, hydrogen serves as an energy storage medium. This capability is vital for balancing the intermittent nature of renewable energy sources like wind and solar power. By storing excess energy in hydrogen form, we can ensure a stable supply even during periods of low energy generation.
Hydrogen is not just a transitional technology; it represents a dynamic component of a sustainable and resilient energy future. Transitioning to a hydrogen economy could thereby become a core strategy for achieving long-term climate goals.
Challenges in Hydrogen Energy Adoption
The adoption of hydrogen energy presents several challenges that must be addressed to facilitate its emergence as a dominant energy source. The significance of these challenges is evident because they directly affect the feasibility and scalability of hydrogen technologies. Understanding these barriers is crucial for researchers and policymakers aiming to promote hydrogen as a sustainable energy solution. This section will focus on two major areas: infrastructure requirements and public perception and acceptance.
Infrastructure Requirements
Infrastructure is a critical component of hydrogen energy systems. The current energy infrastructure is predominantly designed for fossil fuels, making it ill-suited for large-scale hydrogen integration. Transitioning to hydrogen will necessitate the development of new infrastructure or retrofitting existing systems. Key elements include:
- Hydrogen Production Facilities: To meet demand, facilities such as electrolyzers and steam methane reforming plants must be increased in number and efficiency.
- Storage Solutions: Hydrogen has low energy density when stored, so robust storage solutions, including compressed and liquid hydrogen tanks, are essential.
- Transport Networks: Pipelines for hydrogen distribution provide a means for efficient delivery. However, special materials and designs are required to handle hydrogen’s unique properties.
"The infrastructure challenges for hydrogen adoption require innovative engineering solutions to support large-scale projects."
Public Perception and Acceptance
Public perception plays a crucial role in the successful adoption of hydrogen technologies. Several factors influence how individuals and communities view hydrogen, including:
- Safety Concerns: Hydrogen is highly flammable, which raises fears about leaks and explosions. Public education is vital to mitigate these concerns and promote safe practices.
- Misunderstandings about Climate Benefits: Many people may not understand the environmental advantages of hydrogen compared to fossil fuels, particularly if it is produced from renewable sources.
- Economic Interests: Stakeholders in fossil fuel industries may resist hydrogen adoption due to perceived threats to their economic interests, influencing public opinion negatively.
Engaging with communities, transparent communication, and education campaigns can help align public perception with the benefits of hydrogen energy.
Case Studies of Hydrogen Implementation
The exploration of hydrogen implementations provides vital insights into the practical application of hydrogen energy. These case studies reveal not just specific advancements, but also the lessons and considerations that come with diverse endeavors in harnessing hydrogen for electricity generation. Successful projects serve as proof of concept, showcasing the potential of hydrogen, while examining failures helps refine future plans and policies.
Successful Projects and Initiatives
Many projects globally illustrate the successful use of hydrogen as a viable energy source.
- Hydrogen-Powered Trains in Germany: Alstom's Coradia iLint, the world’s first hydrogen passenger train, debuted in Lower Saxony in 2018. The train operates on hydrogen fuel cells, producing no harmful emissions. This project has prompted discussions on integrating hydrogen into various transport sectors.
- FUTURE in Austria: A large-scale green hydrogen production project, FUTURE, utilizes renewable energy to produce hydrogen via electrolysis. The produced hydrogen supports steel production at Voestalpine, showcasing how hydrogen can facilitate industrial decarbonization.
- Hydrogen Fuel Cell Buses in California: Several transit authorities have adopted hydrogen fuel cell buses to reduce carbon emissions. The California Air Resources Board has funded projects where these buses operate in urban centers, demonstrating a cleaner alternative to diesel.
The success of these projects highlights the adaptability of hydrogen technology to various applications, from transportation to industrial processes.
Lessons Learned from Failures
Despite numerous successes, the journey of hydrogen energy is littered with setbacks that provide essential learning opportunities.
- The Hydrogen Highway Initiative: A project in California aimed to create a network of hydrogen refueling stations. Although it started with strong government support, insufficient demand and coordination caused its gradual decline. This highlights the importance of developing infrastructure alongside demand generation plans.
- The Hindenburg Disaster (1937): This tragic event is often cited as a significant setback for hydrogen as a transport fuel. While it was a unique application of hydrogen, the public's perception of safety was profoundly affected. This underlines the need for rigorous safety protocols and public education about hydrogen technology.
- Air Products’ Hydrogen Production Facility in Tennessee: Initially promising, this facility faced economic challenges due to fluctuating natural gas prices. It serves as a reminder that economic viability is critical, and projects must adapt to changing market conditions.
"Case studies of hydrogen implementation serve as a mirror reflecting both the potential and the barriers, laying the groundwork for informed future developments."
Ending and Recommendations
The conclusion of this article serves an instrumental role in framing the significance of hydrogen as a sustainable energy carrier. As we have explored throughout the text, hydrogen offers a remarkable potential to transform energy systems globally. It is critical to recognize not only the scientific and technical aspects but also the social and economic implications associated with this transition.
One of the primary benefits of adopting hydrogen technologies is the potential for a significant reduction in carbon emissions. Hydrogen can serve as a clean and efficient alternative to traditional fossil fuels, particularly in sectors like transportation, industry, and power generation.
Furthermore, recommendations for future action include greater investment in hydrogen infrastructure, research and development into more efficient production methods, and fostering public-private partnerships. These steps will accelerate the integration of hydrogen into mainstream energy systems and enhance energy security.
In summation, hydrogen represents a cornerstone for a sustainable energy future, provided that stakeholders actively pursue the necessary technological advancements and regulatory frameworks.
Summary of Key Insights
In reviewing the main points discussed, several key insights emerge. First, the methods of hydrogen production—ranging from electrolysis to reforming—highlight the versatile means by which hydrogen can be harnessed as an energy source. Each method comes with its own challenges and opportunities, emphasizing the need for tailored approaches in different contexts.
Moreover, the efficiency of hydrogen-to-electricity conversion through fuel cells illustrates its potential as a leading technology in the energy landscape. Understanding the various types of fuel cells, specifically Polymer Electrolyte Membrane Fuel Cells, Phosphoric Acid Fuel Cells, and Solid Oxide Fuel Cells, is crucial for pinpointing their applications and limitations.
The environmental benefits of hydrogen also cannot be overstated. For instance, the mitigation of greenhouse gas emissions bolsters its attractiveness for combating climate change. However, it is essential to balance these benefits by recognizing the economic considerations, particularly in production costs and market dynamics.
Future Research Directions
The exploration of hydrogen systems is still in its infancy, thus providing an array of opportunities for future research. Key areas for investigation include:
- Improving the efficiency of production methods: Researching ways to enhance existing methods like electrolysis and steam methane reforming can lead to lower costs and a smaller carbon footprint.
- Developing advanced fuel cell technologies: Innovations in fuel cell efficiency and durability could significantly broaden the range of applications for hydrogen electricity.
- Understanding the full lifecycle of hydrogen: Evaluating the environmental impact, from production to end-use, will help in policy development and public acceptance.
Moreover, interdisciplinary approaches that integrate economics, engineering, and environmental science are essential for optimizing hydrogen systems. Ensuring that researchers, policymakers, and industry stakeholders collaborate will enhance the overall effectiveness of hydrogen energy initiatives.