Comprehensive Insights into HPLC Spectrophotometers
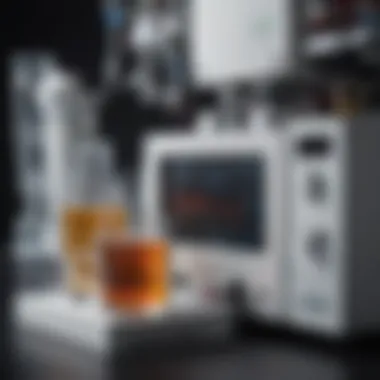
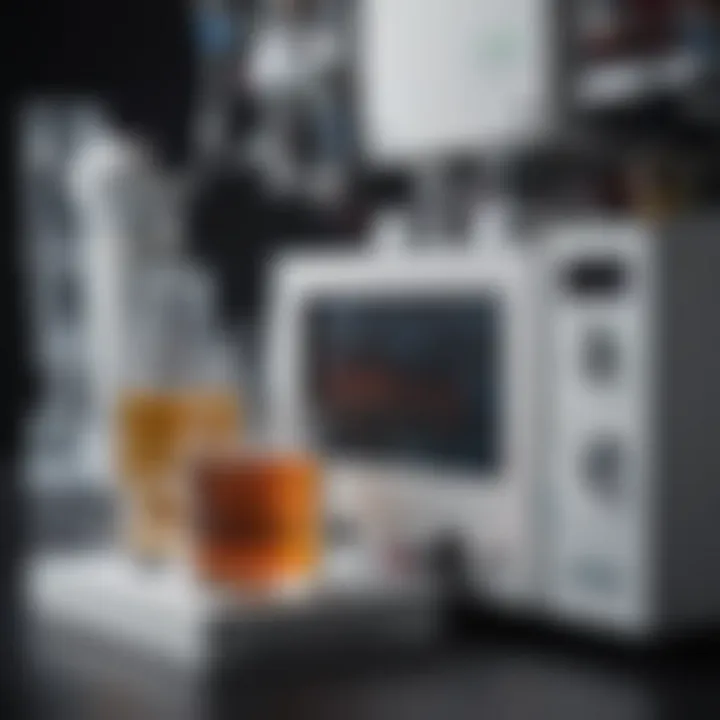
Intro
In the realm of analytical chemistry, High-Performance Liquid Chromatography (HPLC) stands tall as a hero. It allows scientists to separate, identify, and quantify compounds in a mixture, making it an indispensable tool in various fields such as pharmaceuticals, environmental analysis, and food safety. At the heart of this technology lies the HPLC spectrophotometer, a precise instrument that plays an essential role in detecting and quantifying the components separated by chromatography.
When coming across HPLC spectrophotometers, you might wonder about the key concepts that make them tick. This article aims to break down these concepts while delving into the underlying principles, components, and applications of this vital technology. By understanding how HPLC spectrophotometers function, one can appreciate their significance in experimental setups and research initiatives. Let's dig deeper into the fundamentals of HPLC, highlighting why it deserves a closer look.
Key Concepts and Terminology
Definition of Key Terms
To navigate the intricate conversation surrounding HPLC spectrophotometers, familiarizing oneself with a few key terms is crucial:
- Chromatography: This technique enables the separation of components based on their interaction with a stationary phase and a mobile phase.
- Spectrophotometry: Involves measuring how much light a substance absorbs at different wavelengths, providing insights into its concentration.
- Detectors: Components that identify separated compounds as they elute from the chromatographic column.
Concepts Explored in the Article
As we progress through this article, multiple aspects of HPLC spectrophotometers will be examined, including:
- The operational mechanics of the spectrophotometer within an HPLC setup.
- Types of detection methods and their impact on analytical outcomes.
- The technological advancements that have significantly enhanced HPLC's efficiency and accuracy.
- Challenges faced in the field and future perspectives that could shape the evolution of this technology.
In the following sections, we will dissect these concepts methodically, guiding readers through the fundamentals of HPLC spectrophotometers while ensuring a comprehensive understanding of their role in analytical chemistry.
Foreword to HPLC Spectrophotometers
High-Performance Liquid Chromatography (HPLC) has become the bread and butter of analytical procedures in the modern laboratory. For students, researchers, educators, and professionals, understanding HPLC spectrophotometers is crucial for both academic and practical applications. These instruments have significant implications not just in chemistry, but also in pharmaceuticals, environmental science, food safety, and more.
Definition and Importance
HPLC spectrophotometers serve as specialized tools used for separating, identifying, and quantifying compounds that are present in a mixture. This technique is essential for the analysis of complex samples. By using high pressure to push a solvent containing the sample through a column filled with solid adsorbent material, substances are separated based on their different interactions with the stationary phase.
The importance of HPLC spectrophotometers can’t be overstated. They play a critical role in quality control, ensuring that products meet specified standards—something that is non-negotiable in industries like pharmaceuticals. Moreover, they enable researchers to conduct in-depth investigative studies, contributing to scientific advancements.
Historical Background
The journey of HPLC spectrophotometers is quite fascinating. The concept of chromatography dates back over a century to the work of Mikhail Tsvet, who first used the technique in the early 1900s to separate plant pigments. HPLC's modern form emerged in the 1970s when the combination of high-pressure systems with sophisticated detectors revolutionized the landscape of chemical analysis. Unlike traditional chromatography that relied on gravity, HPLC uses pumps to force the mobile phase through the column, allowing for faster processing times and greater efficiency.
Over time, innovations such as the development of various types of detectors—like UV-Vis, fluorescence, and mass spectrometry—have further enhanced the capability of HPLC spectrophotometers being able to detect compounds at incredibly low concentrations, making them invaluable in various research and industrial applications.
Fundamental Principles of HPLC
High-Performance Liquid Chromatography, often abbreviated as HPLC, is a cornerstone of modern analytical chemistry. Understanding the fundamental principles of HPLC is not only vital for the operation of this sophisticated technology but also for interpreting its results accurately. This section breaks down the essential elements that define HPLC's utility, shining a light on how these principles fuel its pervasive use across various scientific endeavors.
Gradient and Isocratic Techniques
These two techniques form the backbone of how HPLC systems are operated. In gradient elution, the composition of the mobile phase gradually changes during the run, allowing for better separation of compounds with varying polarities or other properties. It essentially means that the mix of solvents is tweaked as the analysis progresses, which can enhance the resolution of closely related species.
On the other hand, isocratic elution utilizes a fixed solvent mixture throughout the analysis. This approach is simpler and often less time-consuming. It’s dependable for separating less complex mixtures or when working with highly non-polar compounds. Each method, be it gradient or isocratic, has its distinct advantages and specific scenarios where it truly shines. Making the right choice between these techniques can substantially impact the quality of the analysis and the clarity of the results.
Separation Mechanisms
Separation is not merely a technical term in HPLC; it’s the very essence of the method. The interplay of separation mechanisms defines how effectively components of a mixture can be isolated from one another. Here, we delve deeper into three notable mechanisms: partition, ion exchange, and size exclusion.
Partition
Partition chromatography can be seen as the old reliable of separation techniques. It operates on the principles of differential solubility, which means different compounds dissolve in the mobile phase to varying extents. The key characteristic here is how solutes distribute themselves between two phases: the stationary phase and the mobile phase. Its popularity lies in its effectiveness with a wide range of compounds including pharmaceuticals and natural products.
A unique feature of partition chromatography is the ability to achieve high resolution with complex mixtures. Thanks to varying partition coefficients, even closely related entities can be distinguished. However, it’s not without challenges; the method may rely heavily on solvent choice, and the wrong solvent could lead to negligible separation results.
Ion Exchange
Ion exchange chromatography can be thought of as a matchmaker for charged species. It selectively separates ions based on their charge interactions with the stationary phase. This method is particularly useful in biochemistry for purifying proteins or nucleic acids. The key trait of ion exchange is its ability to target specific ions in a mixture, making it indispensable in areas like pharmaceuticals, where precise identification is crucial.
A unique element of this technique is how it allows for high capacity and specific retention, which can be incredibly advantageous. However, the reliance on ionic strength and pH adjustments proves to be both an advantage and a handicap; while it provides flexibility, it can also complicate method development.
Size Exclusion
Finally, size exclusion chromatography, or gel filtration, employs a rather straightforward concept: separation based on molecular size. The pores in the stationary phase allow smaller molecules to enter while larger ones are effectively excluded, leading to a quick separation based on size. The beauty of this method lies in its simplicity and the non-destructive nature of the approach. Commonly used for separating biomolecules, like proteins and polysaccharides, it’s a popular option in academic and industrial labs alike.
What stands out here is that size exclusion chromatography doesn’t depend on sample polarity, which opens up a wide range of applications. However, one should note that this technique typically doesn’t offer much in terms of resolution compared to its counterparts and can be less effective in complex mixtures.
In summary, understanding these foundational principles of HPLC enables scientists and researchers to tailor their methodologies for specific applications, thereby maximizing both efficiency and analytical accuracy. Knowing when and why to use gradient versus isocratic techniques, or selecting the appropriate separation mechanism, all contribute to the value of HPLC as a powerful analytical tool.
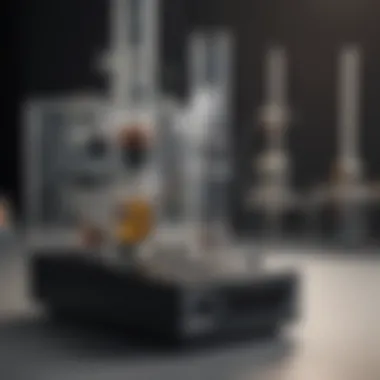
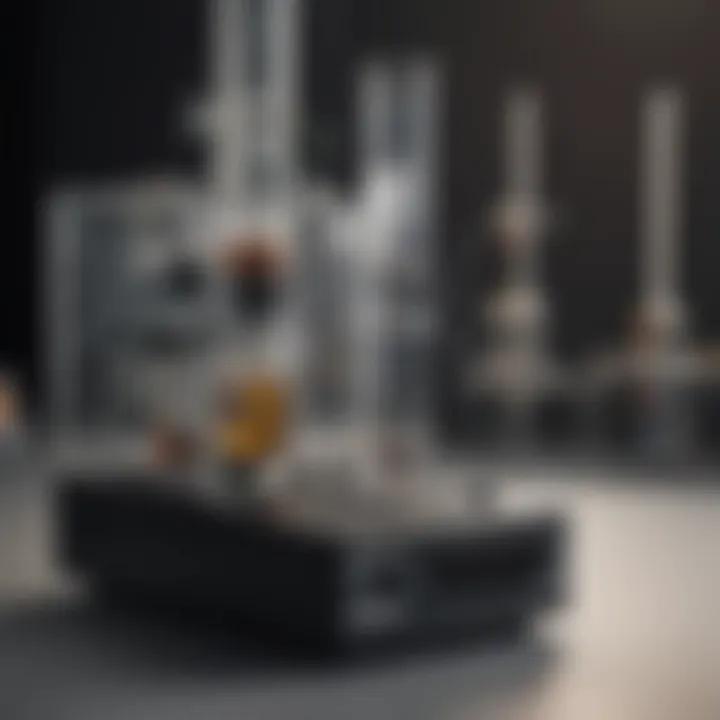
Components of HPLC Spectrophotometers
Exploring the components of HPLC spectrophotometers is cruxial for grasping how they function effectively in various analytical contexts. Each part plays a significant role in ensuring that the process runs smoothly, from the sample introduction to the detection of analyzed compounds. Understanding these elements allows researchers and students alike to appreciate the technical intricacies and innovate on existing methodologies.
Pump Systems
At the heart of any HPLC system are the pump systems, which are indispensable for sustaining the flow of the mobile phase through the column. A well-designed pump ensures that the pressure is consistent while delivering varying flow rates, which is crucial for both gradient and isocratic methods. The importance of stability here cannot be overstated; any hiccup in flow can result in discrepancies in analysis, potentially leading to incorrect conclusions.
Here are a few key characteristics of pump systems:
- Precision: High-performance pumps are designed to maintain precise and reproducible flow rates.
- Durability: Constructed to withstand rigorous conditions, quality pumps have materials that resist corrosion and wear.
However, it is worth noting that they do come with challenges, such as potential leaks which can lead to sample wastage or contamination.
Column Types
The choice of column type directly influences the efficacy of the separation process:
Reversed Phase
Reversed phase chromatography is arguably the most utilized column type in HPLC due to its immense versatility. In this setup, non-polar stationary phases are combined with polar mobile phases, creating a unique environment for separating compounds based on hydrophobicity. This method's major advantages lie in its compatibility with a wide range of analytes, making it a go-to for many applications, including pharmaceuticals and biochemistry.
- Key Characteristic: Robust separation of polar compounds.
- Benefit: Excellent resolution at relatively short run times.
A unique feature of reversed phase systems is that they can handle samples with a broad polarity range, though they do face challenges with highly polar or ionic samples, often requiring specific methods for effective analysis.
Normal Phase
Normal phase chromatography, although less common than reversed phase, holds its ground in certain applications. In this instance, a polar stationary phase is employed alongside a non-polar mobile phase. This setup allows for the separation of non-polar compounds effectively, and it's particularly advantageous for analyzing lipids and organic solvents.
- Key Characteristic: Exceptional for non-polar samples.
- Benefit: Provides a different separation mechanism that can complement reversed phase methods.
However, it's important to consider that this technique can be more complex, requiring careful tuning of solvent conditions to optimize separation outcomes.
Detectors
Finally, the detectors are the eyes of the HPLC spectrophotometer; they intercept and quantify the separated components as they elute from the column.
UV-Vis Detectors
UV-Vis detectors are commonplace in HPLC setups, primarily due to their ability to monitor analytes that absorb light in the ultraviolet and visible spectra. Their significance in the analytical process lies in their robustness and relatively low cost.
- Key Characteristic: Ability to detect a wide range of compounds based on their light-absorbing properties.
- Benefit: Quick response times allow for real-time monitoring of elution.
However, a limitation is that they only detect UV/Vis-absorbing substances, which makes them less suitable for non-absorbing compounds unless additional derivatization steps are implemented.
Fluorescence Detectors
Fluorescence detectors are adored in the field for their sensitivity and specificity. When an analyte fluoresces upon excitation, it provides an incredibly low detection limit, making this method optimal for trace analysis.
- Key Characteristic: Notable sensitivity to low concentrations of analytes.
- Benefit: Offers the potential for quantitative analysis with great precision.
Yet, the main setback is that not all compounds exhibit fluorescence naturally, so often pre-treatment or derivatization is necessary.
Mass Spectrometry Coupling
Mass spectrometry coupling significantly elevates the analytical capabilities of HPLC systems. This integration allows for corroboration of molecular weights alongside separation, offering an edge in complexity handling—especially for biomolecules and complex mixtures.
- Key Characteristic: Dual functionality providing both separation and structural identification.
- Benefit: High sensitivity and specificity, addressing a wide variety of analytical challenges.
The only caveat is that incorporating mass spectrometry can increase the complexity of the setup, demanding a deeper understanding and careful method development from the analyst.
"HPLC spectrophotometers are only as good as their components; mastering these intricacies is key to effective analysis."
Through keen understanding of these components, professionals in analytical chemistry can better navigate the maze of methodologies, ensuring robust and reliable outcomes in their research.
Operating Principles of HPLC Spectrophotometers
Understanding the operating principles of HPLC spectrophotometers is pivotal for anyone delving deep into analytical chemistry. While the technical jargon may sound daunting first glance, grasping these elements can significantly empower researchers and practitioners alike in their efforts. The significance lies in how these devices segregate complex mixtures into their individual components, which ultimately provides critical data for various applications, from pharmaceuticals to food safety.
Sample Injection Techniques
Sample injection is where it all begins. A successful HPLC analysis hinges on how well samples are introduced into the system. Traditional methods include manual injections, using a syringe to load samples directly into the injection port. This approach, although straightforward, does have its drawbacks regarding consistency. In contrast, automatic injection systems provide precision and uniformity; they use peristaltic pumps and syringes for delivering samples, minimizing human error.
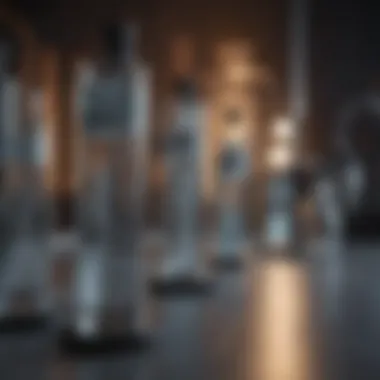
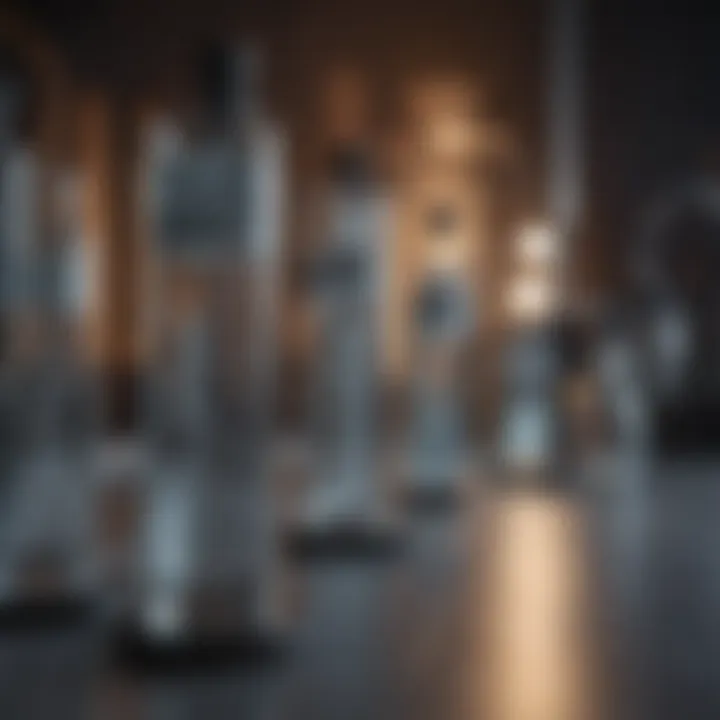
The efficiency of the sample injection technique can greatly influence subsequent results. Incomplete injection may lead to inaccurate quantification, which can skew the analysis. Here are some commonly employed sample injection techniques:
- Loop Injection: Sample is drawn into a loop that then introduces it into the flow path. This method can be highly reproducible.
- Direct Injection: Sample is injected directly into the mobile phase, but it is less commonly recommended due to potential contamination and loss of resolution.
- Solid Phase Extraction (SPE): Ideal for complex matrices, it allows for cleaning samples prior to injection.
Each technique has its merits, and selecting one depends on specific analysis needs including what kind of matrix is being analyzed, sensitivity required, and the overall goals of the experiment.
Mobile Phase Interaction
If the sample injection kicks off the party, the mobile phase is where the action occurs. The mobile phase is the solvent or mixture of solvents that carry the sample through the chromatographic system. Choosing the right mobile phase isn’t just a trivial task; it’s fundamental for successful separations.
Different mobile phases can interact with the samples in unique ways. For instance, in reversed-phase HPLC, non-polar solvents are typically used, leading to stronger interactions with hydrophobic analytes. Conversely, in normal-phase HPLC, polar solvents play a crucial role, often favoring polar compounds.
Interactions in HPLC can generally be classified as follows:
- Hydrophobic interactions: Influencing the elution order of samples based on their polarity.
- Electrostatic interactions: Particularly relevant in ion-exchange chromatography, where charged groups of the stationary phase interact with oppositely charged analytes.
- Van der Waals forces: These minor interactions can also contribute to the overall separation mechanics.
The careful selection of the mobile phase not only affects resolution but also can impact analysis time. A well-optimized mobile phase helps achieve better peak shapes, thereby enhancing sensitivity and improving the reliability of results.
"The mobile phase is like the wind beneath the wings of HPLC; without it, there's simply no flight into the realm of separation."
In wrapping up this section, it’s clear that understanding these operating principles is more than just technical know-how; it’s about creating conditions that facilitate meaningful results. Sample injection techniques and mobile phase interactions are two foundational areas that every user of HPLC technology should be intimately familiar with to navigate complexities in analytical chemistry fluidly.
Applications of HPLC Spectrophotometers
High-Performance Liquid Chromatography (HPLC) spectrophotometers are indispensable tools in modern analytical laboratories. Their applications span diverse fields, reflecting their critical role in achieving accurate and reliable results. Understanding these applications not only highlights their significance but also illustrates the benefits they bring to various sectors.
Pharmaceutical Industry
The pharmaceutical industry relies heavily on HPLC spectrophotometers for drug formulation and quality control. In the development of new medications, these instruments help scientists determine the purity and concentration of active pharmaceutical ingredients (APIs). For instance, when a new drug enters clinical trials, ensuring that its formulation meets rigorous safety standards is crucial. HPLC can detect impurities or breakdown products that might compromise drug efficacy or safety, allowing researchers to make necessary adjustments before proceeding.
Key aspects of HPLC in pharmaceuticals include:
- Quality Assurance: Consistently verifying that production batches meet predefined standards helps maintain safety and efficacy.
- Bioavailability Studies: Understanding how well a drug is absorbed in the body is essential. HPLC assists in quantifying drug levels in biological samples, providing insights into pharmacokinetics.
- Method Validation: Each new analysis requires rigorous validation to ensure that it yields accurate and precise results. HPLC protocols undergo this validation process systematically.
Environmental Monitoring
In environmental science, HPLC spectrophotometers play a crucial role in monitoring pollutants and contaminants in air, water, and soil. These instruments can separate, identify, and quantify various substances that may pose risks to human health and the environment. For example, analyzing water samples for pesticide residues ensures that drinking water remains safe for the population.
Applications in environmental monitoring can include:
- Detection of Hazardous Chemicals: Identifying the presence of harmful substances such as heavy metals or organic toxins allows for timely intervention.
- Ecological Studies: HPLC aids in studying the impact of industrial discharges on aquatic life by monitoring chemical concentrations.
- Regulatory Compliance: Industries must adhere to environmental regulations, and HPLC aids in ensuring compliance by providing necessary data on emissions and waste products.
Food and Beverage Analysis
In the food and beverage sector, HPLC is utilized for various quality control processes, ensuring that products meet safety standards and consumer expectations. It is widely used for analyzing food ingredients, detecting contaminants, and verifying label claims. Notable applications include:
- Nutritional Analysis: HPLC determines the content of vitamins, amino acids, and fatty acids in food products, helping manufacturers ensure they meet regulatory requirements and marketing claims.
- Flavor and Fragrance Testing: In the beverage industry, HPLC is instrumental in identifying and quantifying flavor compounds, ensuring consistent taste in products like juices and wines.
- Food Safety Testing: Detecting adulterants or harmful substances in food products ensures consumer safety and upholds brand integrity.
"Applications of HPLC spectrophotometers underscore their versatility and reliability, making them a cornerstone of analytical chemistry across various industries."
Advantages of HPLC Spectrophotometers
The advantages of HPLC spectrophotometers are essential aspects that set them apart in analytical chemistry. Their unique features contribute greatly to the overall effectiveness and efficiency of various scientific analyses. In this section, we will break down the specific elements of high resolution and sensitivity, as well as their versatility across disciplines. Understanding these advantages is crucial for students, researchers, and professionals engaged in the ever-evolving field of chemical analysis.
High Resolution and Sensitivity
One of the standout characteristics of HPLC spectrophotometers is their high resolution. This distinguishes them from other analytical techniques. The high resolution allows for the precise separation of compounds, even when they are structurally similar. This is a game changer in situations where accuracy is non-negotiable. For instance, in the pharmaceutical industry, when analyzing the purity of drugs, achieving high resolution ensures that contaminants are identified and quantified accurately.
Sensitivity is another pillar of HPLC technology. It refers to the detector's ability to identify even trace amounts of a substance. In practical terms, this means that HPLC can detect concentrations as low as parts per billion. Such sensitivity is invaluable. For example, in environmental monitoring, researchers can pick up harmful pollutants in water samples that other methods might overlook. The ability to discern such low levels of analytes makes HPLC a powerful tool in ensuring public health and safety.
"The capacity to separate and quantify substances at minuscule levels is transformative for research. It pushes the boundaries of what we can discover and measure."
Versatility Across Disciplines
The versatility of HPLC spectrophotometers is truly remarkable. They find applications across a wide range of fields, from pharmaceuticals to environmental science, food safety, and even forensic analysis.
- Pharmaceutical Analysis
- Food and Beverage Industry
- Environmental Testing
- Forensic Science
- In drug formulation, HPLC is crucial for quality control, assessing both purity and potency.
- The capacity to analyze food for contaminants, additives, and nutritional content ensures safety and compliance with health regulations.
- HPLC techniques are used to quantify pollutants and assess environmental health, making them vital for ecological research.
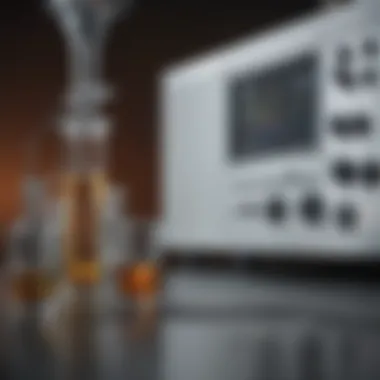
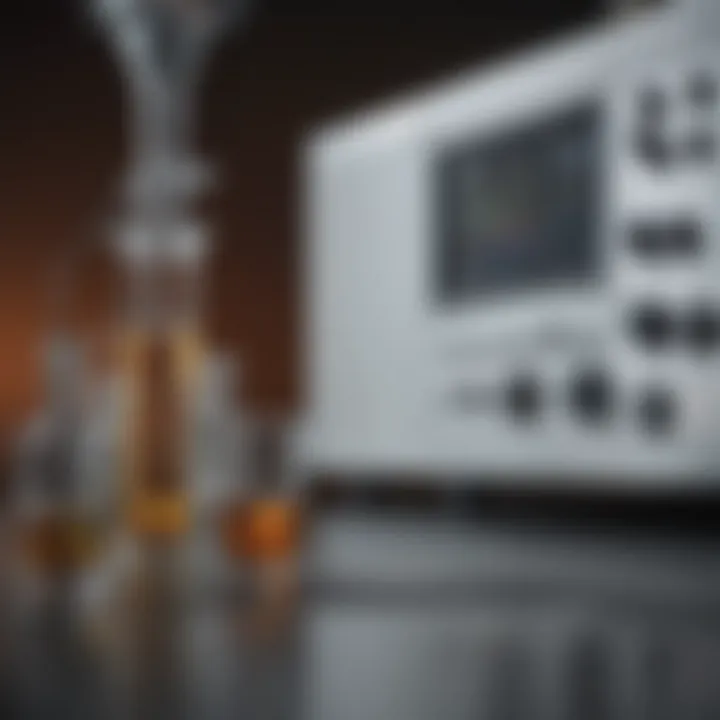
- In criminal investigations, HPLC assists in drug identification and toxicology, thereby playing a key role in legal proceedings.
The adaptability of HPLC systems to various sample types—be it liquids or gels—further enhances its appeal. Users aren't usually hamstrung by the operational constraints that afflict other techniques. This flexibility empowers researchers and professionals in diverse fields to derive meaningful insights from their work.
In summary, the advantages of HPLC spectrophotometers lie in their unmatched resolution and sensitivity, as well as their versatility across a spectrum of applications. These features not only bolster the accuracy and reliability of analyses but also open new avenues for exploration in science and industry.
Challenges in HPLC Analysis
High-Performance Liquid Chromatography (HPLC) is a cornerstone in analytical chemistry. However, despite its powerful capabilities and relevance across various fields, it isn’t without its own share of hurdles. Understanding the challenges in HPLC analysis is crucial for anyone engaging with this technology. From issues associated with sample preparation to the complexities of method development, each aspect can significantly influence the outcomes of analyses. This section aims to delve into these challenges, elucidating their specific elements, benefits, and considerations that researchers must navigate to yield reliable results.
Sample Preparation Complexity
Sample preparation is often the unsung hero in any analytical workflow, yet it’s one area that can lead to significant variability and complications in HPLC results. Proper sample prep not only ensures that the analytes of interest are concentrated enough for detection but also removes any interferences that might skew outcomes. The complexity arises from several factors, including:
- Diversity of Sample Types: Different matrices (like water, soil, or biological samples) present unique challenges in extraction and cleanup processes. Each requires tailored approaches, which can complicate standardization.
- Contaminant Removal: Residual impurities can muddle results. In some cases, analytical chemists may have to maneuver through a maze of filtration, centrifugation, or even solid-phase extraction to achieve clarity in their samples.
- Homogenization Variability: It's vital to ensure that samples are evenly mixed. If one part of the sample is rich in analyte while another is dilute, it can lead to inaccurate readings.
Navigating these intricacies can be time-consuming and requires a meticulous approach. There’s a certain weight on the shoulders of researchers in ensuring that their preparation techniques are robust and repeatable, which can often feel like walking on eggshells.
Method Development Issues
Method development is another critical phase in HPLC analysis that doesn’t come without its troubles. Even seasoned professionals can find themselves neck-deep in intricacies when figuring out the right parameters for their methods. Some of the common hurdles include:
- Parameter Optimization: Finding the perfect combination of parameters — like flow rates, column temperatures, and mobile phase compositions — is tricky. A slight tweak can send results spiraling or, worse, render the process altogether ineffective.
- Reproducibility: An essential characteristic of any analytical method, reproducibility can suffer if conditions aren’t controlled meticulously. Variations in even minor details can lead to inconsistent results, leading researchers down a rabbit hole of re-evaluation.
- Regulatory Standards: Adhering to quality assurance protocols and regulatory requirements in method validation poses additional challenges. It’s not just about finding a working method; researchers must ensure it can stand up to scrutiny in regulated environments, complicating the process further.
As the saying goes, "the devil is in the details," and it certainly holds true in the realm of HPLC method development. Each choice in this stage can have domino effects on the data quality, and thus, attention to detail is paramount.
"Ensuring rigorous methods and precise sample preparation can often make the difference between success and failure in HPLC."
Future Trends in HPLC Technology
The realm of High-Performance Liquid Chromatography (HPLC) is continuously evolving, and understanding the future trends in HPLC technology sheds light on where the field is headed. Staying informed about these trends is crucial for students, researchers, and professionals who rely on HPLC for their work. With ongoing advancements, new applications, and integrating cutting-edge technologies, the advantages become evident. Not just efficiency, but enhanced accuracy and a wider range of detection capabilities stand to benefit analytical chemistry as we know it.
Miniaturization Techniques
Miniaturization in HPLC tools is a game-changer. Smaller devices, such as micro and nanoscale HPLC, allow for the analysis of minute samples, a feat essential in fields like drug development or environmental testing, where only trace amounts may be available.
This enables a range of benefits:
- Reduced Sample Volume: Researchers no longer need large quantities of samples, which is particularly useful for precious or rare materials.
- Higher Throughput: Smaller systems tend to facilitate quicker analyses, so multiple samples can be processed in a shorter span of time.
- Less Reagents: The minimized volume means lower costs and reduced waste, aligning with sustainable lab practices.
Miniaturization doesn’t merely streamline processes; it may also unveil new pathways in analyses that were previously too cumbersome or impossible with traditional equipment. As mentioned, shifting from larger apparatus to miniaturized forms enhances versatility in research and opens doors to previously unexplored avenues in analytical chemistry.
Integration with Other Analytical Methods
The integration of HPLC with other analytical methods symbolizes the future where hybrid technologies dominate. By combining HPLC with mass spectrometry, for example, researchers harness the strengths of both techniques while overcoming individual limitations.
Such integrations offer myriad advantages:
- Enhanced Sensitivity: Bringing together techniques increases detection limits and allows for more sensitive analyses.
- Comprehensive Data: By integrating methods, the scope of data collected is more robust and informative, ideal for complex analysis such as metabolomics or proteomics.
- Facilitated Analysis: Methods like HPLC with Nuclear Magnetic Resonance (NMR) can streamline workflows, saving valuable time.
Moreover, approaches such as hyphenated techniques enable researchers to achieve improved resolution in mixture analyses. This can be significant in quality control or pharmacokinetics, where precision is paramount. As various methods continue to blend, the depth of analysis results will also elevate, fostering advancements that promote greater understanding within many scientific domains.
In summary, the trends toward miniaturization and integration signify not just a shift in technology, but a considerable leap in capabilities for HPLC spectrophotometers. As the future unfolds, staying attuned to these trends will empower researchers to push the boundaries of what's achievable in analytical chemistry.
Closure
The conclusion of this article sheds light on the significance of HPLC spectrophotometers in the broader context of analytical chemistry and scientific research. Summing up the previously discussed points, it serves as a reflective passage that reiterates the core contributions these instruments make to various fields including pharmaceuticals, environmental studies, and food safety. The crisp resolution and sensitivity they offer fundamentally enhance the reliability of analytical outcomes.
In this exploration, several crucial elements have come to the forefront:
- Critical Role in Analysis: HPLC spectrophotometers are indispensable in achieving precise and repeatable results. Their ability to separate complex mixtures and identify individual components with accuracy cannot be overstated.
- Integration of Technologies: The merging of HPLC with other analytical techniques is paving the way for streamlined methods that not only reduce analysis time but also enhance the quality of data obtained.
- Future Innovations: As the field progresses towards miniaturization and increased automation, it becomes evident that HPLC instruments will continue to evolve, offering improved features and overcoming existing challenges.
Highlighting these points underscores how essential HPLC spectrophotometers are to scientific integrity. They don't just provide results; they underpin the decision-making processes in critical applications that affect the health and safety of populations.
Summary of Key Points
In reflecting on this comprehensive journey through the world of HPLC spectrophotometers, a few key points stand out:
- Definition and importance: Their role in analytical chemistry is pivotal, enabling the separation and quantification of compounds.
- Fundamental principles: Key operating techniques such as gradient elution and varied separation mechanisms impact the choice of methods used.
- Component overview: The intricacies of the pump systems, column types, and detection methods utilized are central to effective analysis.
- Applications across sectors: From pharmaceuticals to food safety, their versatility is evident.
- Challenges faced: Issues like sample preparation and method development necessitate ongoing adjustment within the analytical framework.
- Future directions: The trends toward innovation showcase the dynamic nature of this technology, promising even greater efficiencies ahead.
Final Thoughts on HPLC Spectrophotometers
As the curtain closes on this examination of HPLC spectrophotometers, it's clear that their impact on analysis transcends mere functionality. They embody the advancement of technology that constantly refines our understanding of materials and their behaviors through precise measurements.
One might consider HPLC spectrophotometers not just as tools, but as essential allies in the quest for knowledge in the sciences. They represent the bridge between uncertainty and clarity, guiding researchers to make informed decisions. The future holds much promise, with improvements in capacity and collaboration with emerging technologies standing at the forefront of analytical capabilities.
"HPLC spectrophotometers are like the unsung heroes of laboratories; their silent operation leads to breakthroughs that resonate loudly in the scientific community."
With each paragraph turned, it becomes increasingly evident that the relevance of these tools will continue to shine brightly in the landscape of modern analytical chemistry.