Materials Science and Engineering: Key Insights and Trends
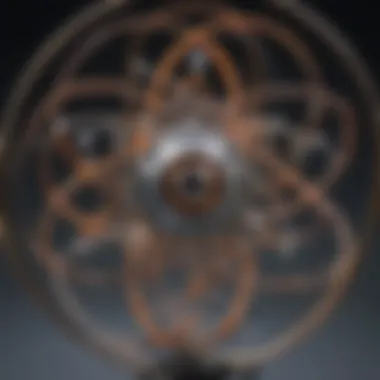
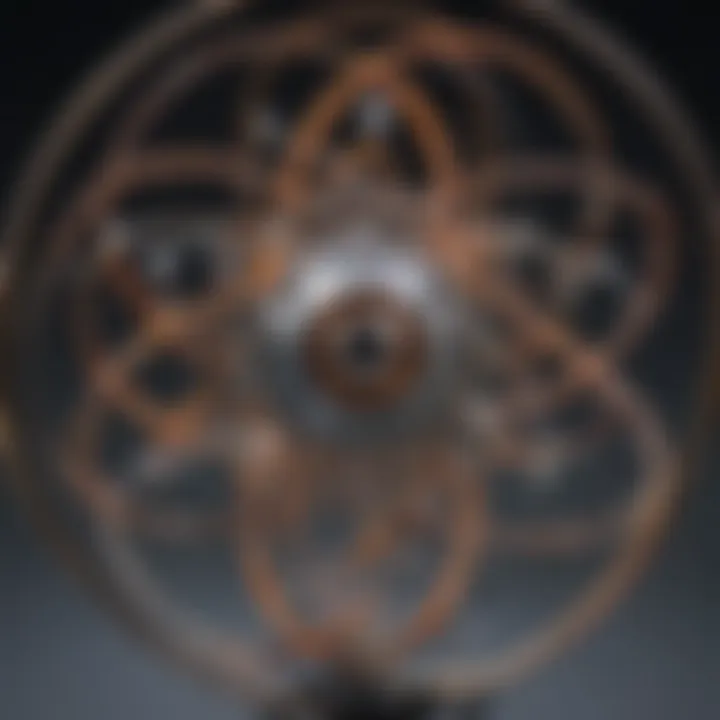
Intro
Materials science and engineering sits at the intersection of multiple disciplines—the fuse of physics, chemistry, and engineering. Its influence can be seen in everything from the smartphone in your pocket to the advanced structures of aerospace vehicles. This field dives into layers that unravel the complexities of materials, shaping the technological world we live in. Understanding these materials is not just a scientific exploration but also a key driver of innovation, performance, and sustainability across numerous industries.
Key Concepts and Terminology
In order to grasp the subject matter fully, it is essential to define a few critical terms and concepts associated with materials science and engineering.
Definition of Key Terms
- Materials Science: The study of materials and their properties, including the relationships between their structure, composition, and performance.
- Materials Engineering: The application of materials science principles to the development and optimization of materials for specific uses in engineering applications.
- Microstructure: The structure of a material observed under a microscope, revealing grain and phase distributions that determine its properties.
Concepts Explored in the Article
- Classification of Materials: Differentiating materials into categories such as metals, ceramics, polymers, and composites. Each category possesses unique characteristics that enable various applications.
- Structure-Property Relationships: Understanding how the arrangement of atoms and molecules within materials influences their physical and chemical properties. This relationship is crucial for tailoring materials for desired applications.
- Materials Selection: The strategic process by which engineers choose materials that meet design and performance criteria, factoring in cost, availability, and performance attributes.
Findings and Discussion
In exploring the breadth of materials science and engineering, several key findings emerge from both historical and contemporary perspectives.
Main Findings
- Evolution of Materials: The timeline showcases humanity's progression from natural materials to advanced alloys and nanomaterials, highlighting how technological advancements and societal needs drive this evolution.
- Role of Advanced Technologies: Innovations such as 3D printing and nanotechnology have drastically changed the landscape, enabling the creation of tailored materials with exceptional properties that were previously unattainable.
Potential Areas for Future Research
The quest for innovative materials continues to be vital for tackling contemporary challenges. Future research may explore:
- Sustainability in Materials: Investigating biodegradable materials and recycling processes to mitigate environmental impacts.
- Smart Materials: Developing materials that respond dynamically to external stimuli, thus opening new avenues for applications in healthcare, electronics, and robotics.
The convergence of materials science with modern technologies is a cornerstone for future innovations, providing pathways that may revolutionize various industries.
In summary, a comprehensive exploration of materials science and engineering reveals a complex, ever-evolving field rich with potential and innovation. By understanding the key concepts, findings, and future directions, students, researchers, and industry professionals can position themselves to contribute meaningfully to this vital discipline.
Understanding Materials Science and Engineering
Materials science and engineering is a cornerstone of modern technology, bridging the gap between diverse scientific disciplines such as physics, chemistry, and engineering. It's more than just the study of materials; it examines how materials are created, how they behave under various conditions, and how they can be engineered for specific applications. In our everyday lives, the implications of materials science are all around us, influencing everything from the smartphones in our pockets to the skyscrapers that define our skylines.
One of the key benefits of understanding materials science is the ability to innovate. New materials lead to innovative solutions that can address contemporary issues—think about the lightweight composites used in aerospace or the heat-resistant ceramics in automotive engines. The field provides not just a lens to look at existing technologies but a toolkit to create the next generation of materials that could potentially revolutionize industries.
Moreover, by comprehending the definitions and scope of materials science, enthusiasts and professionals alike can appreciate the deeper connections between material properties and their applications. This awareness can enhance decision-making processes in various domains, from product design to safety assessments. This comprehensive overview aims to illuminate these aspects, offering insights that are both theoretical and practical.
Definition and Scope
Materials science is fundamentally about understanding the relationship between the structure of materials and their properties. This field spans a wide variety of materials, including metals, ceramics, polymers, and composites. Each category has distinct characteristics that make it suitable for certain applications, be it in construction, medicine, or electronics.
The scope extends far beyond just studying current materials. It encompasses the discovery and development of new materials, processes for manufacturing them, and ways to use them effectively. For instance, researchers actively explore nanomaterials, which offer unprecedented properties due to their minuscule size—often providing enhanced strength, lighter weight, and improved electrical performance.
Interdisciplinary Nature of the Field
The interdisciplinary nature of materials science means that it draws upon principles from a variety of fields. Physics provides insights into the fundamental behaviors of atoms and molecules, chemistry enables the creation of new compounds, and engineering brings those compounds into real-world applications. This collaboration fosters a diverse environment where innovation can flourish.
Interestingly, the interdisciplinary approach enriches not only research but education too. Students in materials science programs often find themselves engaging with different disciplines, conducted through group projects or interdisciplinary courses. This exposure prepares them for real-world challenges where multiple domains intersect.
- Some areas of interdisciplinary connection include:
- Nanotechnology: Utilizing physics and chemistry to create materials at the nanoscale.
- Biomaterials: Combining biology and chemistry to develop materials for medical applications.
- Energy Storage Solutions: Integrating materials science with electrical engineering to improve batteries and capacitors.
This interconnectedness is not just beneficial; it’s essential in tackling complex problems that require a multifaceted approach. As we advance, the integration of fields will continue to diverge and expand, leading to groundbreaking advancements in materials science and engineering.
"Materials science is more than a field; it’s an evolving dialogue between different scientific languages and applications that allows us to redefine what's possible."
By emphasizing the understanding of materials science and engineering, we open doors to myriad possibilities that affect our daily lives and the future of technology.
Historical Development of Materials Science
The historical development of materials science is a fascinating journey through time, showcasing how humans have interacted with various materials to meet their needs and advance their societies. Understanding this evolution is critical for grasping how contemporary materials are utilized and innovated today. The study of ancient materials not only reveals the ingenuity of early civilizations but also sets the stage for modern advancements in technology and engineering.
Ancient Materials and Their Uses
The earliest applications of materials date back to the Stone Age when humans utilized shale and flint to craft tools. These materials, while primitive, were crucial; flint, for instance, was honed to create sharp edges for cutting and scraping. Fast-forward a few milennia, and we see the advent of metalworking. Copper became a game-changer, paving the way for the Bronze Age when a mixture of copper and tin was used to create stronger tools and weapons. This period marked a significant leap in technology, demonstrating the influence of material properties on the capability of tools.
Diverse ancient societies like the Egyptians employed materials like limestone and granite to construct monumental structures. These ancient constructions not only stand as a testament to human creativity and engineering acumen but also illustrate the understanding of material properties like durability and workability.
The Industrial Revolution's Impact
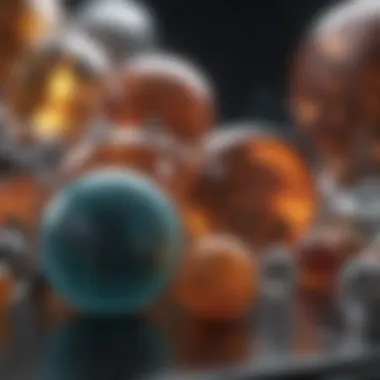
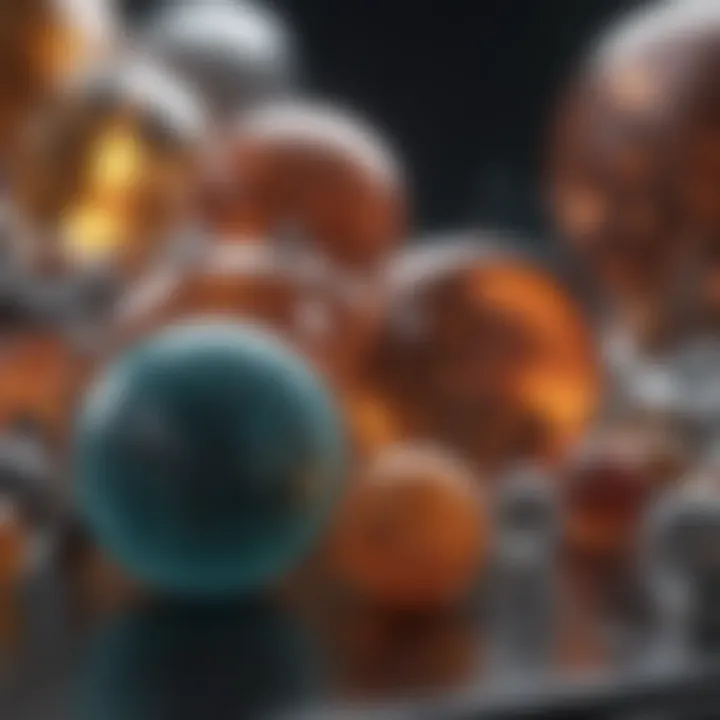
The Industrial Revolution brought about seismic shifts in material usage and creation. The transition from handicrafts to mass production necessitated the development of new materials. Iron, with its abundance and malleability, became a cornerstone material. The introduction of the Bessemer process allowed for the efficient production of steel from molten pig iron, which fundamentally revolutionized construction, machinery, and transportation.
This revolution also saw the rise of ceramics in practical applications, as improved techniques allowed for stronger and more versatile components. The incorporation of other materials, such as rubber and glass, came about through innovative manufacturing processes, expanding the range of applications in industry and daily life. Materials began to be engineered for specific attributes, setting the groundwork for modern materials science.
"The revolution in material availability and innovation during this period reshaped economies and societies around the globe."
Emergence of Modern Materials Science
By the mid-20th century, materials science began to take on a more formal identity as an interdisciplinary field. The discovery of polymers, for example, opened new avenues in material options, leading to applications we now take for granted, from plastics in everyday items to complex composites used in aerospace and automotive industries. Scientists started acknowledging the importance of molecular structure, leading them to explore areas like crystallography and nanotechnology.
The development of advanced characterization techniques allowed scientists to understand materials at an atomic level, leading to further innovations. For example, understanding the details of how materials behave under stress has led to stronger and lighter materials in aviation.
As we dive deeper into the 21st century, the study of materials continues to thrive and adapt. Concepts like sustainability and eco-friendliness are garnering attention, driving research into biodegradable materials and recycling techniques.
In sum, the historical development of materials science serves as a lens through which we can appreciate the strides made by humankind in engineering and innovation, leading us to the sophisticated materials we use today.
Classification of Materials
Understanding how materials are classified is fundamental in the field of materials science and engineering. The classification helps to simplify complex interactions inherent in different materials. By categorizing materials into distinct groups, one can better analyze their properties, applications, and interactions with various environments. This understanding is vital for engineers and researchers who need to select the appropriate material for specific applications.
Why Classification Matters:
- Ease of Understanding: Classifying materials provides a framework that is easier to grasp. It allows professionals to quickly identify the characteristics and potential uses of different materials.
- Optimized Material Selection: Proper classification enables the selection of the best materials for various applications—be it in building a bridge or creating a new electronic device.
- Tailored Performance: Each category comes with a unique set of properties, which can be leveraged to meet the specific demands of any project.
"The right material can make the difference between success and failure in engineering projects."
Now, let's delve deeper into the specifics of each major classification.
Metals and Alloys
Metals are known for their ability to conduct electricity and heat. They are typically strong, ductile, and malleable materials. Alloys, on the other hand, are formed by mixing two or more metals, or a metal with another element, improving certain properties. This enhancement can lead to increased strength, reduced weight, or improved resistance to corrosion.
- Common Metals: Aluminium, Copper, Titanium.
- Popular Alloys: Steel (Iron and Carbon), Brass (Copper and Zinc), Bronze (Copper and Tin).
The selection of metals and alloys is crucial in industries like aerospace and automotive, where weight and strength are critical.
Polymers
Polymers are large molecules made from many repeating subunits; think of them as chains formed from smaller links. They can be natural, like rubber, or synthetic, such as polyethylene or nylon. Their unique properties—such as flexibility, lightweight design, and durability—make them irreplaceable in various applications ranging from packaging to high-performance materials.
- Types of Polymers:
- Thermoplastics (e.g., Polypropylene)
- Thermosetting Plastics (e.g., Epoxy)
- Elastomers (e.g., Natural Rubber)
Polymers are particularly significant in consumer goods; they can be tailored for specific uses, resonating with evolving demands in today’s market.
Ceramics
Ceramics encompass a wide range of inorganic, non-metal materials, which are often brittle but resistant to heat and wear. They are typically composed of metallic and non-metallic elements. Their formation often involves a high-temperature process, resulting in materials that maintain their integrity at elevated temperatures.
- Types of Ceramics:
- Traditional Ceramics (e.g., Pottery, Tiles)
- Advanced Ceramics (e.g., Silicon Nitride, Zirconia)
Ceramics play a crucial role in items needing heat resistance, like insulators in electronic devices and components in jet engines, making their classification imperative for applications dealing with extreme conditions.
Composites
Composites are materials made from two or more constituent materials with significantly different physical or chemical properties. When combined, they produce a material that is superior to the individual components alone. The most notable feature of composites is their incredible strength-to-weight ratios, which makes them particularly attractive in aerospace and automotive applications.
- Common Examples:
- Fiber Reinforced Plastics (e.g., Carbon Fiber composites)
- Concrete (aggregate + cement)
Understanding composites allows for innovative solutions in design, enabling the creation of lightweight yet durable products. This classification reflects the ongoing evolution within materials science, emphasizing tailored engineering solutions.
In summary, classifying materials is a vital exercise in materials science and engineering. It not only aids in understanding properties and applications but also plays a significant role in material innovation and optimal selection processes.
Key Concepts in Materials Science
Materials science is a field that intertwines subjects like chemistry, physics, and engineering to explore the nature of materials and their properties. Understanding key concepts is essential for both practitioners and learners in the field, as these principles influence every stage from design to application. Grasping these fundamentals not only spurs innovation but also helps in selecting the right materials for specific functions, ensuring performance and sustainability. That said, this section outlines critical elements—structure-property relationships, thermodynamics of materials, and kinetics and phase transformations—that form the backbone of materials science.
Structure-Property Relationships
The relationship between a material's structure and its properties is fundamental. To put it plainly, the way a material is arranged at the atomic or molecular level significantly impacts how it behaves in practical scenarios. For instance, metals like steel have a crystalline structure that gives them strength and durability, making them excellent for construction. In contrast, glass, with its disordered structure, is brittle and fragile.
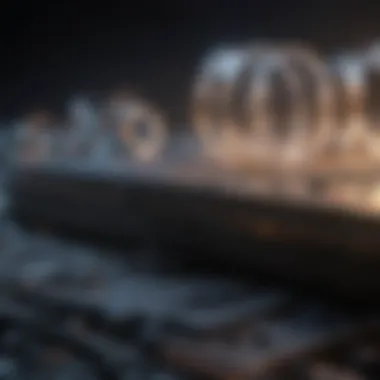
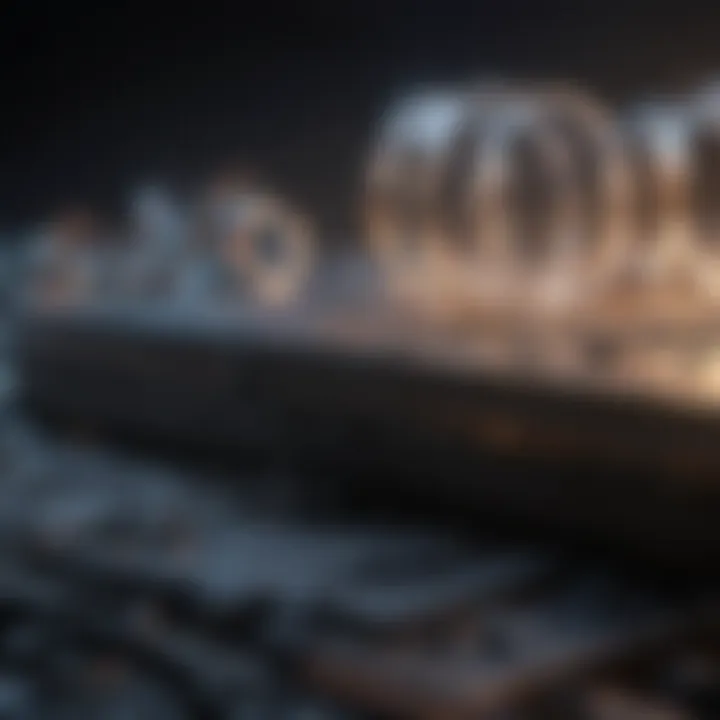
- Atomic Arrangement: The specific arrangement of atoms can affect conductivity. Materials with tightly packed structures often display greater electrical conductivity, making them fit for electronic applications.
- Phase Diagrams: Understanding these diagrams is crucial for materials selection. They illustrate the stability of phases at various temperatures and pressures, guiding engineers in making educated decisions when combining different materials.
In essence, recognizing how a material's microstructure correlates with its macroscale properties allows for better predictive capabilities in material performance.
Thermodynamics of Materials
Thermodynamics is where physics meets materials science, helping to explain energy transformations that occur in materials during processing or application. This concept is not merely theoretical; it manifests in various real-world contexts. Take, for example, the melting temperatures of alloys which depend largely on the thermodynamic properties of individual components.
Factors include:
- Gibbs Free Energy: This indicates the feasibility of phase changes. A lower Gibbs free energy can suggest a more stable phase under given conditions, which is essential for processes like alloying or heat treatment.
- Enthalpy and Entropy: These parameters affect reactions and material stability. An understanding of enthalpy—energy content—and entropy—degree of disorder—can guide engineers in creating materials with desirable properties.
Grasping these thermodynamic principles aids in predicting material behavior under varying conditions, which is paramount for designing processes and materials.
Kinetics and Phase Transformations
While thermodynamics helps in understanding if a reaction can happen, kinetics is about how fast it occurs. This section covers how temperature, pressure, and time influence materials’ phases during processing and application.
- Diffusion: A critical process in phase transformations. For example, in the hardening of steel, carbon atoms diffuse through iron to form cementite, thus altering hardness and wear resistance.
- Nucleation and Growth: These terms describe how new phases emerge during solidification or cooling processes. The rate at which such transformations occur plays a significant role in determining final material properties.
Understanding kinetics exposes the various pathways that materials can take, providing insights into manufacturing efficiency and the resulting material qualities.
"A strong understanding of the key principles in materials science enables innovation in design and engineering, ultimately benefiting countless industries."
Overall, honing in on these key concepts allows practitioners to make informed choices, pushing the boundaries of technology while ensuring the reliability of materials in various applications.
Materials Selection and Design
Materials selection and design occupy a crucial space in the realm of materials science and engineering. Properly selecting materials ensures performance longevity and cost-efficiency in various applications, from mega construction projects to delicate electronics. When you throw various materials into the mix, it’s not just about aesthetics or availability; it’s about how well a material meets specific functional demands while remaining economically viable. A well-thought-out selection process can lead to innovations or improvements in product performance, significantly affecting the overall success of a project.
When considering materials selection, several factors must be analyzed. These include mechanical properties, environmental impact, lifecycle costs, and other specific use-case requirements. It is not merely picking a material off the shelf based on instinct or availability; it’s about alignment with performance specifications and long-term objectives.
Criteria for Material Selection
In choosing a material, engineers and designers assess numerous criteria. Here are some notable considerations:
- Mechanical Properties: Tensile strength, ductility, hardness, and fatigue resistance greatly influence a material’s suitability in stressing situations.
- Thermal Properties: How a material behaves under temperature changes determines its applicability in various environments.
- Corrosion Resistance: In environments where moisture or chemicals are present, a material's resistance to deterioration is vital.
- Cost-Effectiveness: Budget constraints often dictate what is feasible. A cheaper alternative might not always yield better value in the long run if it results in higher maintenance costs.
- Availability and Sustainability: Assessing how easily a material can be sourced and if it aligns with sustainability goals is getting more attention in today’s eco-conscious world.
These criteria are essential, but they can vary based on the specific application. A material that’s perfect for aerospace engineering may not suit the medical industry.
Role of Computer-Aided Design
In the modern engineering landscape, the role of computer-aided design (CAD) cannot be understated. CAD software enhances the material selection process and design development by enabling detailed visualization and simulation. With CAD, designers and engineers have the power to rigorously assess the impact of various materials on a design before any physical prototypes are created.
Some advantages include:
- Enhanced Visualization: Designers can see how the material interacts with other components in real time, allowing for adjustments before anything is physically made.
- Simulation and Testing: Programs can simulate stress, fatigue, and thermal responses, providing insights into how materials perform under different scenarios without any risk.
- Rapid Prototyping: Engineers can quickly iterate new designs and selections, speeding up the development cycle.
- Sustainability Analysis: Many CAD tools now feature modules for environmental impact assessments, which help designers consider the ecological consequences of their material choices.
"Understanding how materials interact within a design is key to solving engineering challenges effectively. CAD systems provide a unique opportunity to explore this efficiently."
Testing and Characterization of Materials
Testing and characterization of materials are foundational elements in materials science and engineering. Understanding how materials perform under various conditions helps researchers and engineers select suitable materials for specific applications. This area of study is not just about finding out what a material is made of; it's about grasping its functionality and durability when used in the real world. By implementing effective testing methods, we can extrapolate critical insights into the behavior of materials, thus enabling innovation in design and engineering.
Mechanical Testing Methods
Mechanical testing involves evaluating the mechanical properties of materials, such as strength, ductility, hardness, and fatigue resistance. These tests are paramount for determining a material's ability to withstand loads and deform under stress.
Common Mechanical Tests
- Tensile Testing: Measures how much a material can be stretched before breaking. The results give insight into tensile strength and elongation.
- Compressive Testing: Shows how materials behave under compressive forces, essential for applications in construction and automotive industries.
- Hardness Testing: Assesses resistance to localized plastic deformation, providing an idea of wear resistance.
- Impact Testing: Determines a material's ability to absorb energy during deformation; it’s crucial for assessing safety in applications like automotive chassis.
Each of these tests provides a different piece of the puzzle, informing engineers which materials are best suited for various applications while ensuring safety and efficacy in real-world scenarios.
Thermal and Electrical Properties
Understanding the thermal and electrical properties of materials is crucial for applications that involve heat transfer or electrical conductivity. This knowledge is especially significant in electronic devices and energy systems.
Key Aspects
- Thermal Conductivity: It measures how well a material can conduct heat. For example, metals such as copper offer high thermal conductivity, making them suitable for heat sinks.
- Electrical Conductivity: This property outlines how well a material conducts electricity. For instance, materials like silver and gold excel in electrical applications due to their low resistance.
- Thermal Expansion: Different materials expand and contract with temperature changes, influencing how they perform in varying thermal environments.
Testing these properties enables engineers to build more efficient systems, assess potential failure points, and create solutions that can withstand varying environmental conditions.
Structural Characterization Techniques
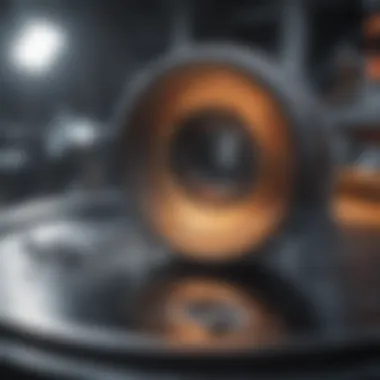
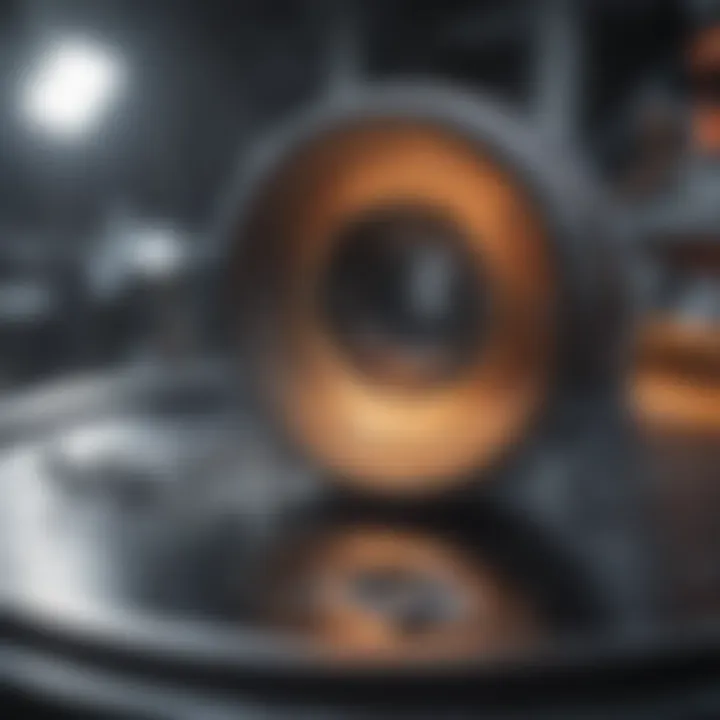
Structural characterization techniques are vital for comprehending the arrangement of atoms in materials and how this affects their macroscopic properties. The right characterization technique can reveal not just how a material looks, but how it behaves under different conditions.
Common Techniques
- X-ray Diffraction (XRD): Utilized for identifying crystalline structures and phase compositions, crucial for materials like metals and ceramics.
- Scanning Electron Microscopy (SEM): Offers high-resolution images of material surfaces, allowing researchers to inspect surface morphology in great detail.
- Transmission Electron Microscopy (TEM): Provides insights into nanoscale properties, which can influence the mechanical and thermal behavior of materials.
By applying these techniques, scientists and engineers can achieve a deeper understanding of fundamental components, enhancing the development of new materials that meet specific criteria for future innovations.
In summary, the testing and characterization of materials serve to bridge the gap between theoretical understanding and practical application, facilitating advancements in technology and engineering.
Current Trends in Materials Science and Engineering
Materials science and engineering continue to evolve at a remarkable pace, influenced by advancements in technology and the pressing needs of society. Understanding these current trends is crucial for students, researchers, and professionals as they navigate the contemporary landscape of this dynamic field. By recognizing the importance of these trends, stakeholders can leverage knowledge to drive innovation and address emerging challenges. The convergence of materials science with other disciplines, such as computing and environmental studies, is reshaping how materials are designed, used, and recycled.
Nanomaterials and Their Applications
The emergence of nanomaterials has redefined what’s possible in materials science, offering unique properties due to their minuscule size. Materials engineered at the nanoscale often exhibit remarkable characteristics like increased strength, reduced weight, and enhanced chemical reactivity. In electronics, nanomaterials facilitate the development of faster and more efficient components. A prime example is carbon nanotubes, which are showing promise in various applications for transistors and other devices.
In the medical field, nanoparticles are making waves in drug delivery systems, enabling targeted therapy that improves the effectiveness of treatments while minimizing side effects. This selective approach contributes considerably to personalized medicine. Furthermore, nanomaterials enhance properties in products as varied as cosmetics, sporting goods, and even food packaging, pushing the boundaries of practicality and safety.
However, the integration of nanomaterials into everyday life comes with challenges. Issues related to toxicity and environmental impact necessitate thorough research and regulation. It’s essential that advancements in nanotechnology are accompanied by responsible management to protect human health and the environment.
Smart Materials and Adaptive Systems
Smart materials, often referred to as "intelligent materials," respond to their environment in real time, adapting to changes around them. They are engineered to exhibit specific properties based on external stimuli, such as temperature, pressure, or electric fields. This exciting realm of materials science has applications that can fundamentally alter various industries.
For instance, shape memory alloys can return to a predefined shape when subjected to a certain temperature. This characteristic finds applications in medical devices, robotics, and construction. Similarly, piezoelectric materials can convert mechanical stress into electrical energy, which opens doors for energy harvesting in various applications, like sensors or smart highways that generate energy as vehicles pass.
Moreover, integrating smart materials into infrastructure leads to self-healing systems. Structures embedded with these materials are capable of detecting damage and initiating repair processes autonomously. While still in its infancy, this technology holds the potential to greatly enhance durability and reduce maintenance costs for everything from bridges to pipelines.
Sustainability and Green Materials
The pursuit of sustainability in materials science has never been more significant. As industries face increasing pressure to adopt environmentally friendly practices, the focus has shifted toward green materials and sustainable production processes. These materials are designed to minimize environmental impact, often using renewable resources and reducing waste.
Bio-based polymers, for example, offer a compelling alternative to conventional plastics derived from fossil fuels. Made from natural sources such as corn or sugarcane, these biodegradable materials are helping to mitigate pollution. Similarly, the development of sustainable composites that incorporate recycled content is gaining traction in construction and automotive sectors, lessening the reliance on virgin materials.
Another aspect of sustainability is life-cycle analysis, which examines the total environmental impact of a material throughout its lifespan, from production to disposal. This holistic approach enables organizations to make informed choices that promote sustainability in materials selection.
"The shift towards sustainability in materials science is not just a trend; it's a response to the urgent need for environmental stewardship."
As the focus on sustainability continues to evolve, it is imperative for the next generation of materials researchers and engineers to embrace these principles, ensuring that future advancements contribute positively to society and the planet.
Future Directions in Materials Science
The future of materials science is not merely a continuation of past practices; rather, it holds the potential for revolutionary changes that could reshape industries and environments alike. A comprehensive understanding of upcoming advancements can aid researchers, students, and professionals in grasping the intricate layers of our material world. Here, we unearth two significant facets of future trajectories in this field: emerging technologies and the solutions they may provide for global challenges.
Emerging Technologies
In the sphere of materials science, technology is advancing at breakneck speed. This rapid evolution is not just about enhancing existing materials, but also about the creation of entirely new classes of materials. Advancements in nanotechnology, for instance, permit the engineering of materials at the atomic or molecular scale. By manipulating materials at such a granular level, we can craft substances with properties tailored for specific applications, be it in medicine, electronics, or construction.
Moreover, the rise of artificial intelligence and machine learning is changing how materials are discovered and optimized. Computers can analyze vast datasets to predict material behaviors, hence significantly cutting down the time from conceptual research to application. A recent example can be found in the development of shape-memory alloys, which can return to a predetermined shape when heated. The integration of AI in their design has accelerated this process, promising robust applications in industries ranging from robotics to biometrics.
Global Challenges and Materials Solutions
As our planet faces a myriad of challenges, materials science has a pivotal role to play in formulating viable solutions. Climate change, resource depletion, and waste management are some of the pressing issues necessitating innovative materials practices.
For example, research is increasingly focusing on the concept of biodegradable polymers, which offer alternatives to conventional plastics that plague our oceans and landfills. These materials can decompose naturally, significantly reducing environmental impact. In addition, the development of recyclable composites can pave the way for a circular materials economy where end-of-life products are reincarnated into new materials, rather than tossed aside.
Investments in sustainable materials that are both economically feasible and environmentally friendly will not only mitigate detrimental effects but may also create new economic opportunities. Scientists are exploring the use of bio-based materials, derived from renewable resources, which could replace petroleum-based products.
"The role of materials science is critically important as we navigate an era defined by global changes and challenges. Innovations in materials can be the key stepping stones leading to sustainable solutions."
Closure
The conclusion of this article serves not simply as an end, but as a vital synthesis of the myriad insights and principles covered throughout our exploration of materials science and engineering. Understanding this field is crucial because it is intricately woven into the very fabric of our daily lives, influencing everything from the design of a smartphone to the infrastructure of skyscrapers. Materials science resonates with notions of innovation and improvement, driving advancements in technology and sustainability.
Summary of Key Insights
In summarizing the key insights from the previous sections, it's clear that this discipline encompasses a wide-ranging scope. One major takeaway is the interdisciplinary nature of materials science, bridging gaps between physics, chemistry, biology, and engineering. Here are some highlighted points:
- Interdisciplinary Integration: Materials scientists often collaborate across domains, showcasing the need for diverse perspectives in solving complex challenges.
- Historical Context: The evolution of materials reflects human ingenuity, from ancient practices to cutting-edge technologies. The lessons from the past guide present-day innovations.
- Classification and Properties: Understanding different material types—metals, polymers, ceramics, and composites—along with their unique properties, is foundational for materials selection and application.
- Emerging Trends: As technologies evolve, new materials emerge, such as nanomaterials and smart systems, which promise to redefine functionality and efficiency in numerous applications.
"In the world of materials science, every discovery opens doors to new possibilities, transforming what we thought was achievable."
Call for Continuous Learning
As we find ourselves in an age characterized by rapid technological advancements, the call for continuous learning in materials science and engineering resonates profoundly. The landscape is constantly changing, with new research, materials, and methodologies emerging regularly. Engaging with this ongoing education is not just beneficial—it is essential.
To stay at the forefront of the field, professionals and aspiring scientists should:
- Participate in Workshops: Hands-on experiences facilitate a deeper understanding of the materials and techniques at play in the field.
- Engage with Online Resources: Platforms like Reddit and Facebook host vibrant communities discussing the latest breakthroughs and challenges in materials science.
- Read Latest Research: Keeping up with journals and articles ensures awareness of innovations and findings that could influence ongoing projects.
- Collaborate Interdisciplinarily: Partnering with experts from other fields can yield fresh insights and approaches.
In closing, that the world of materials science and engineering holds significant promise for the future. It beckons to those eager to learn and innovate, creating solutions that look beyond today and envision the transformative potential of what materials can achieve.