In-Depth Study of Plate Tectonics and Its Effects
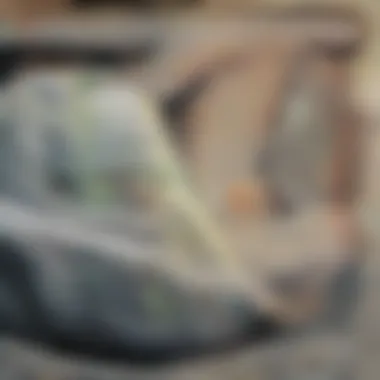
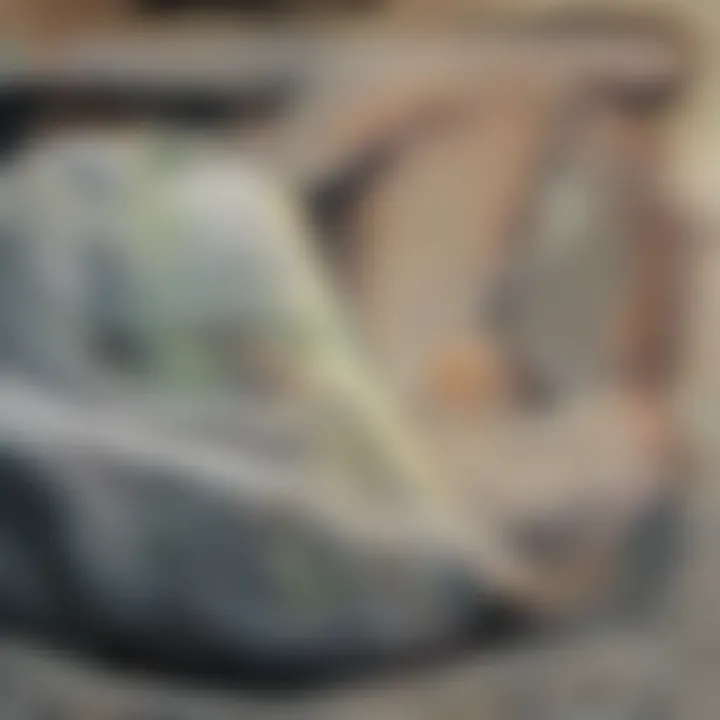
Intro
Plate tectonics is a cornerstone theory in the field of geology. It elucidates the structure and dynamic processes of the Earth's lithosphere. This examination of plate tectonics extends beyond mere definitions; it encapsulates the myriad interactions that govern the behaviors of tectonic plates. The insights into plate boundaries, mechanisms of movement, and resultant geological outcomes are crucial for understanding phenomena such as earthquakes and volcanic eruptions.
The significance of this exploration cannot be understated. As the Earth continues to shift and move, understanding these tectonic processes offers profound implications for predicting natural disasters and grasping the evolution of our planet's structure. Therefore, a comprehensive understanding of this topic is essential for students, researchers, educators, and professionals who seek to enrich their knowledge in geology and related sciences.
Key Concepts and Terminology
Definition of Key Terms
Within the framework of plate tectonics, several key terms need to be defined to facilitate clarity:
- Lithosphere: The rigid outer layer of the Earth, comprising the crust and upper mantle.
- Tectonic Plates: Large, rigid pieces of the Earth's lithosphere that move over the weaker asthenosphere below.
- Plate Boundaries: The interfaces where two tectonic plates meet; categorized into divergent, convergent, and transform boundaries.
- Subduction Zone: An area where one tectonic plate moves under another, often associated with volcanic activity.
- Seismic Activity: The frequency and intensity of earthquakes that occur in a specific area.
Concepts Explored in the Article
The article will delve into various concepts:
- Types of plate boundaries.
- The driving forces behind tectonic movements.
- Geological phenomena linked to plate tectonics, including earthquakes and volcanic eruptions.
- The broader implications of plate tectonics in understanding geological history and predicting future geological events.
Findings and Discussion
Main Findings
The examination of plate tectonics reveals several key findings:
- Plate Movement: Plates drift at approximately 1 to 10 centimeters per year, influenced by mantle convection, slab pull, and ridge push.
- Earthquake Occurrence: Most earthquakes are concentrated along plate boundaries, highlighting the importance of understanding these areas for disaster preparedness.
- Volcanic Activity: Volcanic eruptions often occur at convergent and divergent boundaries due to the interaction of tectonic plates.
Potential Areas for Future Research
In light of ongoing research in tectonics, several avenues emerge for exploration:
- The impact of human activities on tectonic movements, particularly in urban areas prone to seismic activity.
- The role of subduction zones in shaping coastal landforms and their response to climate change.
- Advanced methods for predicting the timeline of major tectonic events and their associated risks.
Understanding plate tectonics is essential for predicting changes in the Earth's crust and mitigating the impacts of natural disasters.
Through systematic study of these findings, the relevance of plate tectonics becomes increasingly clear. The theory not only explains the mechanics of Earth’s structure but also significantly influences our understanding of geological history and future developments.
Intro to Plate Tectonics
The study of plate tectonics is vital for understanding the dynamic nature of the Earth. It provides insight into how the surface of our planet is shaped and transformed over time. This theory is not only fundamental in geology but also essential for related fields such as environmental science and urban planning. Its principles illuminate the interactions between the Earth's rigid plates, leading to various geological phenomena, including earthquakes and volcanic eruptions.
Definition and Historical Context
Plate tectonics refers to the scientific theory that describes the large-scale motions of Earth's lithosphere, which is divided into tectonic plates. These plates float atop the semi-fluid asthenosphere. The concept emerged in the early 20th century when Alfred Wegener proposed the theory of continental drift, suggesting that continents were once connected but drifted apart over geological time. This foundation paved the way for the modern theory of plate tectonics. Over decades, significant research has provided evidence confirming plate movements, including mapping of ocean floors and data from seismic activities.
Scientific Contributions
The advent of plate tectonics has led to profound advancements in various scientific disciplines. It has redefined our understanding of geology and has influenced research in areas such as seismology, volcanology, and ecology. Noteworthy contributions include the studies that revealed the mid-ocean ridges as divergent boundaries, where tectonic plates move apart, and the identification of convergent boundaries leading to mountain formation and trench creation. Understanding these processes enables researchers to predict geological events. Furthermore, the application of plate tectonic theory has significantly improved our ability to assess natural hazards, equipping policymakers with crucial information for disaster preparedness.
Structure of the Earth
Understanding the structure of the Earth is crucial for comprehending plate tectonics. The Earth is composed of distinct layers, each playing a significant role in geological processes. The interactions among these layers are what drive tectonic movements and, subsequently, geological phenomena such as earthquakes and volcanoes. Through examining these layers, one can gain insightful perspectives on how they influence the dynamics of the lithosphere. This knowledge is necessary for students, researchers, and professionals in geology and related fields.
Layers of the Earth
The Earth consists of three primary layers: the crust, the mantle, and the core. Each layer has properties that contribute to tectonic dynamics.
Crust
The crust is the outermost layer of the Earth, varying in thickness from about 5 km under oceans to about 70 km beneath mountain ranges. It represents a thin layer yet is vital for our understanding of plate tectonics. A key characteristic of the crust is its rigidity, providing a stable surface for life. This stability makes the crust a compelling choice for the study of geological activity.
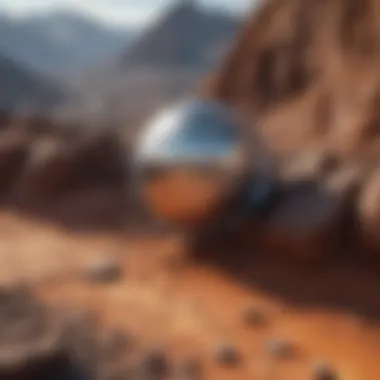
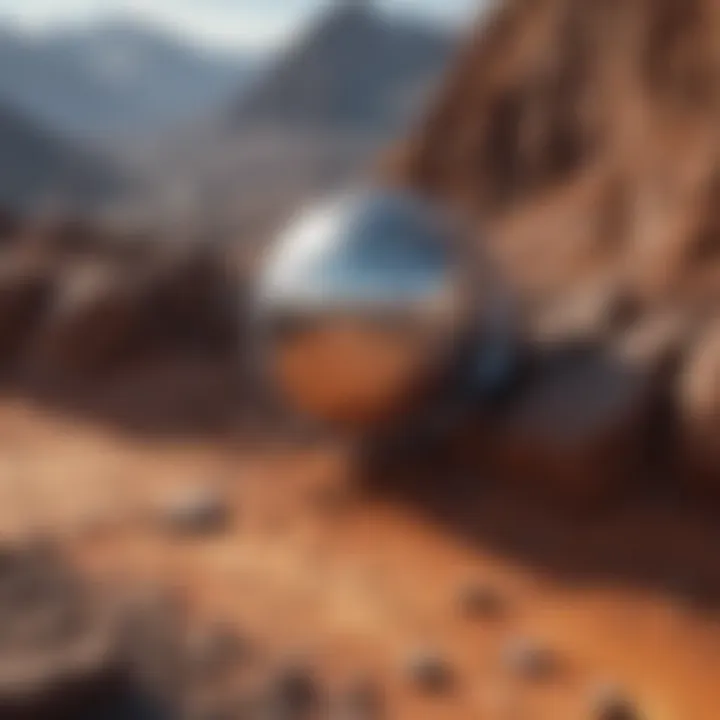
One unique feature of the crust is its composition, which includes a variety of rocks such as granite and basalt. The benefits of studying the crust include understanding its role in forming various landforms and its susceptibility to earthquakes. However, it can be more challenging to study compared to deeper layers due to its accessibility.
Mantle
Below the crust lies the mantle, which extends to a depth of about 2,900 km. The mantle is composed of semi-solid rock that can flow under pressure and heat. Its key characteristic is its convection currents, which play a significant role in driving tectonic plate movements. The mantle's ability to flow makes it a beneficial aspect of this article as it helps explain the mechanisms of mantle convection.
A unique feature of the mantle is the presence of the asthenosphere, a less rigid zone that allows for movement. This feature is advantageous as it directly relates to plate tectonics, impacting how plates interact at boundaries. Understanding the mantle enriches knowledge about geological processes but raises challenges in studying its behavior due to its inaccessibility.
Core
The core is the innermost layer of the Earth, composed primarily of iron and nickel. The core is divided into the outer core, which is liquid, and the inner core, which is solid. A critical characteristic of the core is its role in generating the Earth’s magnetic field through its convective movement. This aspect is significant as it adds to the overall understanding of how tectonic activity influences planetary features.
Its unique feature lies in its temperature, reaching up to 5,700 degrees Celsius. This extreme heat contributes to the motion of the molten outer core, affecting tectonics. Studying the core can provide insights into Earth’s formation but poses challenges regarding accessibility and data collection.
Lithosphere and Asthenosphere
The lithosphere consists of the crust and the upper part of the mantle, forming the rigid outer shell of the Earth. Its strength and rigidity are essential for understanding the behavior of tectonic plates. In contrast, the asthenosphere lies beneath the lithosphere and is characterized by its semi-fluid properties. This difference in characteristics plays a crucial role in the movement and interaction of tectonic plates, influencing various geological phenomena.
In summary, the detailed examination of the Earth's layers is fundamental to grasping the principles of plate tectonics. Each layer has distinctive attributes that together explain the processes shaping our planet's surface.
"Understanding the structural components of the Earth is vital for deciphering the complex interactions that dictate geological movements."
Types of Plate Boundaries
Understanding the types of plate boundaries is crucial in the field of geology. This knowledge provides insights into the dynamic processes that shape our Earth. Plate boundaries are the regions where two tectonic plates meet, and their interactions lead to significant geological phenomena. By classifying these boundaries, scientists can predict geological activity, assess risks of natural disasters, and understand the nature of the Earth's surface changes. Each boundary type has distinct characteristics and outcomes, influencing everything from mountain formation to earthquake occurrence.
Divergent Boundaries
Divergent boundaries occur where two tectonic plates move apart from each other. This movement creates new crust as magma rises to the surface. A primary example of this is the Mid-Atlantic Ridge, where the Eurasian and North American Plates diverge, forming new oceanic crust. The importance of divergent boundaries lies in their role in seafloor spreading and the creation of ocean basins.
Key points to consider include:
- Creation of New Crust: As plates separate, magma fills the gap, which solidifies to form new crust. This process is essential in continuous material recycling of the Earth’s lithosphere.
- Volcanic Activity: Divergent boundaries often exhibit significant volcanic activity as magma finds its way through fractures. This activity contributes to the formation of underwater volcanoes and volcanic islands.
- Seismic Events: While typically less intense than those at convergent boundaries, earthquakes can occur at divergent boundaries as the plates pull apart and create tension.
Convergent Boundaries
Convergent boundaries arise when two tectonic plates collide. The interaction at these boundaries can lead to one plate being forced beneath the other in a process known as subduction. This type of boundary is associated with some of the most powerful earthquakes and volcanic eruptions on the planet, particularly along the Pacific Ring of Fire.
Aspects of convergent boundaries include:
- Landforms: The collision of plates can give rise to mountains, like the Himalayas formed by the collision of the Indian Plate and the Eurasian Plate.
- Subduction Zones: These areas mark where more dense oceanic plates sink beneath lighter continental plates. This is crucial for the recycling of oceanic crust back into the mantle.
- High Seismic Activity: The stress from the collision results in frequent earthquakes. The intensity of these quakes can be significant, potentially causing widespread damage.
Transform Boundaries
Transform boundaries are characterized by the lateral sliding of tectonic plates past one another. Unlike divergent and convergent boundaries, transform boundaries do not create or destroy crust. Instead, they cause frictional forces that can lead to significant earthquakes. The San Andreas Fault in California is a classic example of a transform boundary.
Important features of transform boundaries include:
- Earthquake Generation: The sliding of plates can cause stress accumulation which is released as earthquakes. These events can occur unexpectedly and can be quite destructive.
- Limited New Landform Creation: Generally, transform boundaries do not create new landforms like mountains or oceanic ridges but can produce fault lines visible on the surface.
- Geological Study Importance: Understanding transform boundaries helps predict seismic risks and develop safety protocols in populated regions.
"Plate boundaries are not only crucial for understanding tectonic activity but are also essential in assessing natural disaster vulnerabilities."
In summary, the types of plate boundaries—divergent, convergent, and transform—play a pivotal role in shaping the geological landscape of the Earth. The interactions at these boundaries lead to a variety of geological phenomena, offering profound implications for natural disaster preparedness, environmental assessments, and continued geological research.
Mechanisms Driving Plate Movements
Understanding the mechanisms driving plate movements is essential for comprehending how the Earth's lithosphere interacts and evolves. These mechanisms reveal the underlying processes that facilitate tectonic activity. They explain not only the movement of the tectonic plates but also the consequences of such movements, including earthquakes, volcanic eruptions, and mountain formation. Each mechanism is important, as it provides insights into the dynamics of Earth's interior and surface. Thus, focusing on these processes can enhance predictive capabilities and improve disaster preparedness.
Mantle Convection
Mantle convection is a primary driver of plate tectonics. It involves the slow, circular movements of the mantle's material due to heat transfer from the Earth's core. Hotter material in the mantle rises toward the surface, while cooler material sinks. This circulation creates convective currents that exert forces on the overlying tectonic plates.
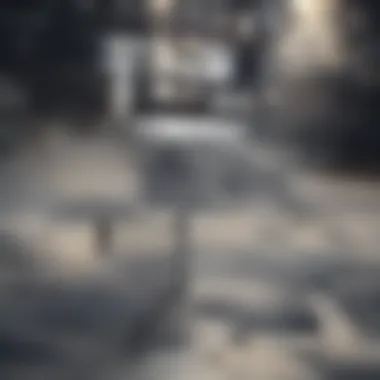
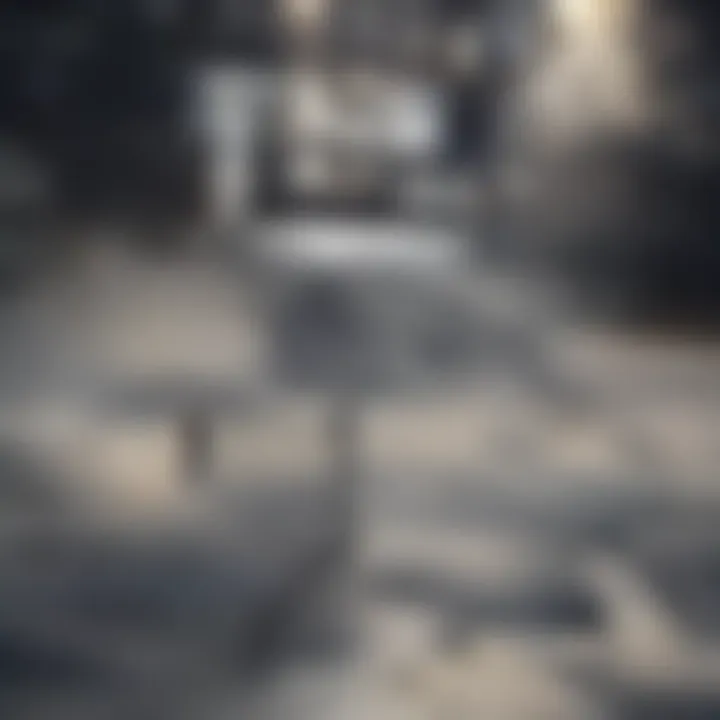
- Heat Source: The heat comes from both radioactive decay and residual heat from the formation of the Earth.
- Movement Patterns: The motion is not uniform; different parts of the mantle can move at varying speeds, affecting how the plates above them shift.
- Influence on Plate Movement: As these currents move, they push and pull plates in different directions, promoting activities at divergent, convergent, and transform boundaries.
This process is fundamental in driving the continual reshaping of Earth's surface and directly influences geological phenomena.
Slab Pull and Ridge Push
Slab pull and ridge push are two critical concepts that explain specific forces acting on tectonic plates.
- Slab Pull: This phenomenon occurs at subduction zones, where one plate sinks beneath another. As the denser oceanic plate descends, it pulls the trailing plate along with it. This mechanism is particularly significant in the movement of tectonic plates. It contributes to the velocity at which plates can move.
- Ridge Push: At mid-ocean ridges, new oceanic crust is created as magma rises to the surface. This new material is hot and less dense, causing it to be elevated relative to the surrounding areas. The gravitational sliding of this elevated ridge pushes the plates apart, further aiding in tectonic movement.
Understanding these mechanisms allows researchers and students to appreciate the complexities of tectonic processes better. They are essential for assessing risks associated with geological hazards, helping to inform mitigation strategies.
Geological Phenomena Associated with Plate Tectonics
Plate tectonics is not just a theoretical framework; it is a critical aspect of the Earth's geology that influences a range of geological phenomena. Understanding these phenomena, such as earthquakes, volcanic activity, and mountain building, provides insight into the dynamic processes that shape our planet. Each of these phenomena arises from the interactions of tectonic plates, and their study is essential for geologists, seismologists, and those involved in disaster preparedness.
Earthquakes
Earthquakes are among the most immediate and dramatic products of tectonic activity. These seismic events occur when stress along faults, caused by the movement of tectonic plates, exceeds the strength of rocks. This results in a sudden release of energy, generating seismic waves that propagate through the Earth. The consequences can be devastating, leading to loss of life and extensive property damage, especially in populated areas.
Earthquakes are classified by their intensity and depth. The scale used to measure their magnitude is the Richter scale, while the Modified Mercalli Intensity scale evaluates the effects on people and structures. Understanding the mechanics behind earthquakes helps in identifying seismic hazard zones, crucial for urban planning and risk mitigation.
In terms of prediction, while exact forecasting remains elusive, advances in seismic monitoring technologies have improved the ability to assess risks. For instance, seismologists often employ the study of historical seismicity to gain a clearer picture of potential future events.
Volcanoes
Volcanic activity is another significant geological phenomenon associated with plate tectonics, particularly at convergent and divergent boundaries. When tectonic plates interact, magma can rise to the surface, resulting in volcanic eruptions. These eruptions might vary from effusive lava flows to explosive eruptions, profoundly altering the landscape and affecting climate.
Volcanoes serve as indicators of tectonic activity. As magma accumulates beneath the Earth's crust, it creates pressure which can lead to eruptions. Monitoring gas emissions and ground deformation are critical for predicting potential volcanic activity. Understanding magma movements also contributes to forecasting possible impacts on surrounding ecosystems and human structures.
Major volcanic events in history, such as the eruption of Mount St. Helens or the eruption of Krakatoa, illustrate the potential catastrophic effects on both local and global scales. Researchers study these events not only to learn about past catastrophes but also to improve future response strategies.
Mountain Building
Tectonic activity also plays a vital role in the formation of mountain ranges. This process, known as orogeny, primarily occurs at convergent boundaries, where two continental plates push against each other. The immense pressure generated leads to folding, faulting, and uplift of Earth's crust, forming mountain ranges like the Himalayas and the Andes.
The study of mountain building involves understanding the geological history of these regions. Geologists examine rock layers, fossil records, and age dating to reconstruct the processes that led to their formation. Additionally, mountain ranges impact local climates and ecosystems, creating distinct environments.
Continental Drift Theory
The Continental Drift Theory is a pivotal concept in understanding plate tectonics. This theory, proposing that continents were once joined together and have since drifted apart, serves as the fundamental basis for many geological principles. Its relevance lies in explaining not just the current positions of continents but also the historical and ongoing processes shaping the Earth's surface. By recognizing the movements of land masses over geological time, researchers can gain insights into past climates, biological distribution, and the formation of various geological features.
Historical Development of the Theory
The genesis of the Continental Drift Theory can be traced back to the early 20th century, where Alfred Wegener, a German meteorologist, laid foundational ideas. In 1912, he introduced the concept in his book "The Origin of Continents and Oceans". Wegener posited that continents were once part of a supercontinent known as Pangaea, which began to break apart around 200 million years ago. His observations included the similarities in fossil records found on different continents and the congruence of various geological formations, like the Appalachian Mountains in North America correlating with the Caledonian Mountains in Scotland.
Despite compelling evidence, Wegener faced considerable skepticism. Critics argued that he lacked a mechanism to explain how continents could drift. Mainstream geologists dismissed his ideas until the advancement of plate tectonics in the 1960s provided the necessary framework. Wegener's contributions, once marginalized, became recognized as transformative. Eventually, the notion of continental drift gained acceptance as the scientific community furnished evidence through plate tectonic theory, explaining the movements through mantle convection and seafloor spreading.
Pangaea and its Significance
The concept of Pangaea is crucial in illustrating the idea behind continental drift. Pangaea represents a hypothetical supercontinent that existed approximately 335 million years ago. Its significance extends beyond mere geography; it holds profound implications for biological diversity and evolution. The separation of landmasses led to isolated ecosystems, fostering unique evolutionary paths. Thus, species that developed in one region often could not migrate to others, contributing to the rich biodiversity observable today.
Geologically, Pangaea's existence explains many features of the Earth's landscape. Mountain ranges, rock formations, and mineral deposits can often be traced back to processes associated with the break-up of this ancient supercontinent.
"Understanding the drift of continents contributes significantly to our comprehension of Earth's physical and biological history."
In terms of ongoing research, exploring continental drift allows scientists to predict potential shifts in ecosystems, mitigate natural disasters, and understand the evolutionary history of species around the globe.
Application of Plate Tectonics in Natural Disaster Preparedness
Understanding plate tectonics is crucial for effective natural disaster preparedness. By grasping the dynamics of tectonic movements, societies can better predict and respond to natural disasters like earthquakes and volcanic eruptions. The application of this knowledge not only enhances disaster response but also informs land-use planning and public policy.
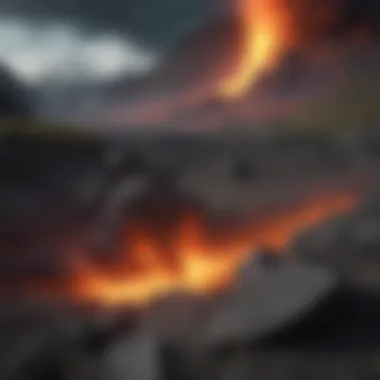
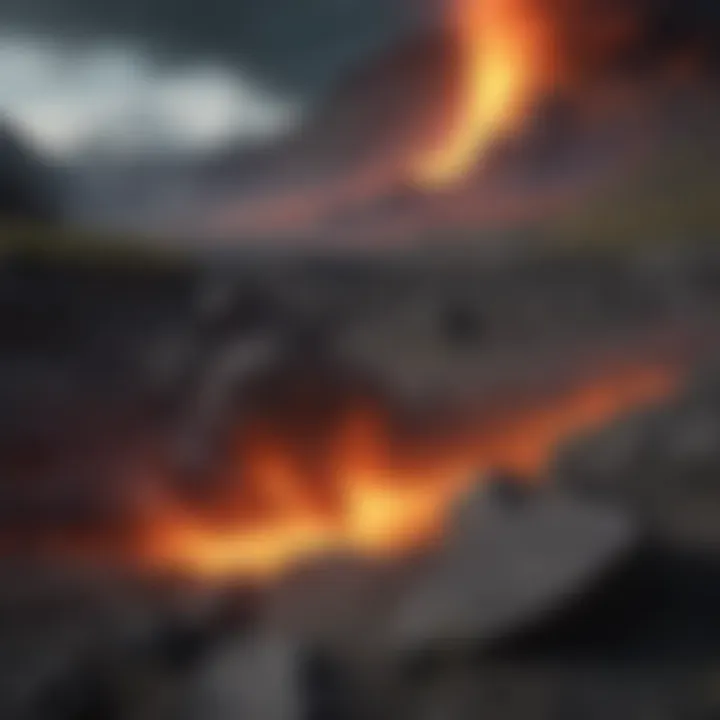
Seismic Hazard Assessment
Seismic hazard assessment is a fundamental element in disaster preparedness. It involves identifying and analyzing areas that are at higher risk for earthquake occurrences. Mapping fault lines, utilizing historical data, and studying tectonic plate boundaries are significant steps in this process. Advanced technologies, such as seismic monitoring networks and satellite imagery, provide real-time data that can improve risk assessment accuracy.
A detailed seismic hazard assessment includes:
- Identifying vulnerable regions: Understanding where faults are located helps in determining which areas are more susceptible to earthquakes.
- Evaluating building codes: Adjusting construction standards based on seismicity ensures that structures can withstand potential shocks.
- Public education: Informing communities about seismic risks promotes preparedness and safety.
A well-conducted seismic hazard assessment can significantly reduce the loss of life and property during seismic events, making it a key component of disaster preparedness strategies.
Risk Mitigation Strategies
Risk mitigation strategies are implemented to minimize the potential impacts of natural disasters related to plate tectonics. These strategies encompass a range of actions designed to lessen vulnerability and enhance resilience in communities situated near tectonic boundaries.
Key risk mitigation strategies include:
- Infrastructure improvements: Strengthening bridges, roads, and buildings to withstand earthquakes is essential. Implementing retrofitting measures can save lives.
- Early warning systems: Developing and maintaining early warning systems can provide critical seconds to minutes of alert prior to seismic activity. This can enable people to take cover and reduce casualities.
- Community drills and planning: Regularly conducting drills helps prepare residents for emergencies, ensuring they know what to do when an earthquake or volcanic event occurs.
- Government policies: Establishing regulations for land use in high-risk areas helps limit the construction of unaffordable structures in dangerous zones.
The integration of risk mitigation strategies informed by plate tectonics enhances community resilience and preparedness. Through proactive planning and implementation, potential disaster impacts can be significantly reduced, ultimately saving lives and preserving property.
"Preparedness is key; understanding the behavior of our planet can help us avoid the worst consequences of natural disasters."
By comprehensively applying the principles of plate tectonics, societies can forge a proactive approach to disaster preparedness, fostering safer environments for all.
Future Directions in Plate Tectonics Research
Exploring future directions in plate tectonics research is vital for understanding how ongoing developments can influence geological science. Research into tectonics impacts various fields such as ecology, climate science, and disaster management. The increase in technological capabilities and theoretical advancements contributes to a richer understanding of tectonic processes and their implications for our planet.
Technology and Methodological Advances
Recent advancements in technology play a crucial role in enhancing the precision and scope of tectonic studies. Tools such as satellite-based GPS measurements enable researchers to monitor plate movements with unprecedented accuracy. This data is essential for developing models that explain tectonic dynamics in real time.
Additionally, seismic imaging techniques provide insights into the Earth's interior structure. By examining seismic waves generated by earthquakes, scientists can inform their understanding of how tectonic plates interact beneath the surface. Advanced computer modeling and simulations are also being utilized to predict tectonic behavior under various scenarios.
"The integration of advanced technology in geological research has revolutionized our approach to understanding Earth's dynamics."
Global Climate Interactions
The interplay between plate tectonics and global climate systems is gaining attention. As tectonic activity can influence atmospheric conditions over geological time, understanding these connections is essential. For instance, volcanic eruptions can release significant quantities of ash and gases into the atmosphere, temporarily altering climate patterns. In turn, climate changes can affect erosion patterns and sedimentation processes, further impacting tectonic activities.
Research in this area focuses on long-term climate data in relation to tectonic events. Studies aim to determine how tectonics contribute to patterns of climate change over millions of years. The implications for current climate models are profound, suggesting a need for integrative approaches that account for tectonic influences amid global warming trends.
Finale
In this article, the conclusion serves as an essential element that encapsulates the vital discussions surrounding plate tectonics. Plate tectonics, as a foundational theory, has profound implications for understanding geological phenomena and the dynamics of the Earth. The exploration of this topic highlights key principles, such as the movement of tectonic plates, the types of boundary interactions, and the associated geological events like earthquakes and volcanoes.
Importance of Understanding Plate Tectonics
Understanding plate tectonics is important for several reasons. Firstly, it enhances our knowledge of Earth's structure and the processes that shape its surface. This comprehension is crucial for predicting geological hazards and assessing risks effectively. For instance, areas along tectonic boundaries are more prone to earthquakes, necessitating thorough seismic hazard assessments. Moreover, understanding these dynamics also leads to better disaster preparedness and response strategies, ultimately saving lives.
Furthermore, the study of plate tectonics informs us about the historical movements of continents and their future trajectories. This knowledge bears significance not only for geologists but also for researchers in climate science, as the interactions between changing plate movements and climatic systems are increasingly recognized.
"Plate tectonics is vital for understanding the Earth’s past and predicting its future trends."
Benefits and Considerations
A profound understanding of plate tectonics also illuminates broader implications related to resource management. Minerals, oil deposits, and even groundwater availability often relate to tectonic processes. Thus, knowledge gained from geological studies impacts economic decisions.
Finally, appreciating the complexities of plate tectonics can foster a respect for our planet's geological systems, emphasizing the need for sustainability and careful stewardship of natural resources. As our environment changes, studying these tectonic processes may reveal insights into future geological and climatic trends, underscoring the ongoing relevance of this field of study.
In summary, the conclusion of this article not only integrates various discussions on plate tectonics but also reinforces its significance. As we move forward, the continuing research in this area will remain critical, not just for geology but for our broader understanding of Earth as a complex system.
Importance of References
- Validation of Information: References serve to validate claims made about plate tectonics, such as the mechanisms involved in plate interactions or the historical context of theories like continental drift.
- Guidance for Further Research: An extensive reference list allows readers to delve deeper into specific topics of interest, enhancing their understanding and encouraging further study.
- Acknowledgment of Contributions: Proper referencing acknowledges the work of other scholars and researchers, fostering a spirit of collaboration within the academic community.
Benefits of a Comprehensive Reference List
- Clarity: Readers can easily follow up on claims and seek more detailed explanations.
- Interdisciplinarity: Sources from diverse fields such as geology, environmental science, and physics can enrich the discussion.
- Current Knowledge: Keeping references updated ensures that the article incorporates the latest discoveries and theories.
Considerations in Choosing References
- Reputation of Sources: Well-established sources such as academic publishers and recognized universities should be prioritized.
- Relevance to Content: Only include sources that enhance or relate directly to the information discussed in the article.
- Date of Publication: Ensure that the references reflect recent advancements in the understanding of plate tectonics.