Understanding Aerodynamics Testing: Key Principles and Techniques
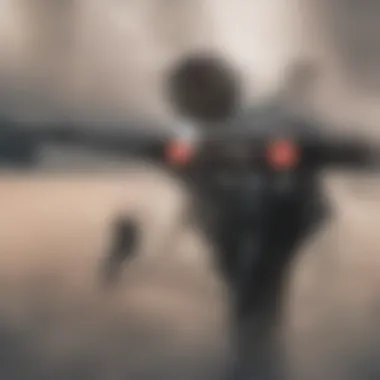
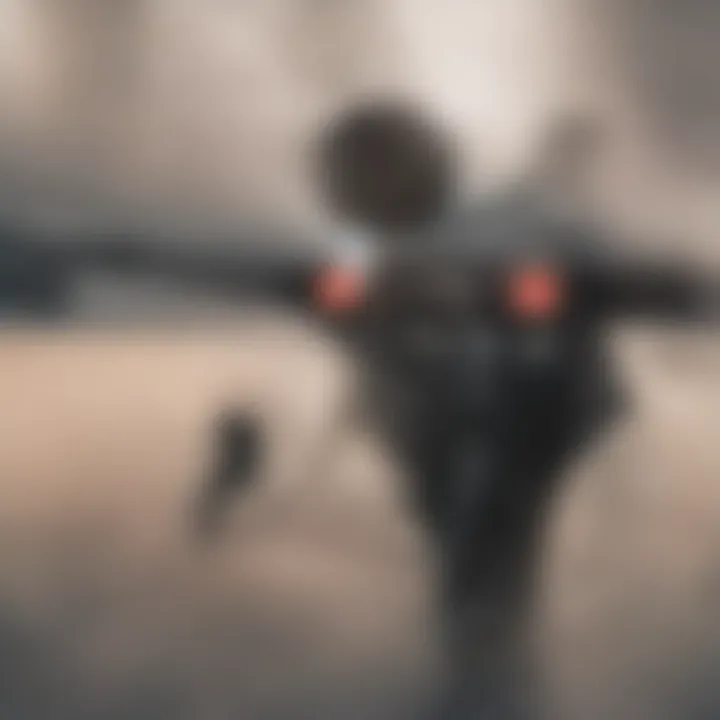
Intro
Aerodynamics testing is a vital area of study that focuses on the behavior of air as it interacts with solid objects. Understanding this interaction can greatly enhance performance in various fields such as aerospace, automotive, and even sports. Insights gleaned from aerodynamics testing have significant implications, influencing everything from aircraft designs to the shape of sports equipment.
The relevance of aerodynamics testing goes beyond theoretical applications. It is instrumental in creating safer, more efficient vehicles and equipment. By exploring the principles, techniques, and practical applications of this study, one can uncover how empirical data influences technology development and safety regulations.
Key Concepts and Terminology
Aerodynamics may appear complex, but breaking it down reveals some fundamental concepts and terminology that are essential for understanding the field.
Definition of Key Terms
- Aerodynamics: The study of how air interacts with moving objects.
- Drag: The resistance experienced by an object moving through air.
- Lift: The upward force acting against the weight of an object.
- Testing techniques: Methods used to evaluate aerodynamic performance, such as wind tunnel testing or computational fluid dynamics (CFD).
Concepts Explored in the Article
This article will cover:
- The basic principles of aerodynamics that underpin testing methodologies.
- Various testing techniques employed in the field.
- Applications across industries, showcasing the importance of aerodynamic analysis.
Findings and Discussion
Main Findings
The examination of aerodynamics testing has revealed critical insights into its role in design and safety. For instance, wind tunnel testing remains a cornerstone of measuring the aerodynamic properties of vehicles and aircraft. It allows engineers to visualize airflow and model potential performance before full-scale production begins.
Computational fluid dynamics is also transforming how researchers understand aerodynamics. This approach provides detailed simulations, reducing the need for extensive physical testing.
Potential Areas for Future Research
Despite the advances made, several areas are ripe for exploration:
- Improved CFD methods that enhance accuracy and efficiency.
- The impact of surface textures on drag reduction.
- The role of aerodynamics in electric vehicle designs.
"Continued research in aerodynamics is essential for the innovations of tomorrow's technologies."
This article aims to provide a thorough understanding of aerodynamics testing. By recognizing its significance in various applications, researchers and professionals can better appreciate the data generated and its implications.
The interplay between theory and practical application forms the backbone of this study, fostering advancements that ultimately lead to improved designs and enhanced safety across many sectors.
Prologue to Aerodynamics
Aerodynamics is a critical field of study that examines the behavior of air as it interacts with solid objects. This topic is particularly important in various engineering disciplines where understanding the principles of aerodynamics can lead to significant advancements. From improving the efficiency of airplanes to enhancing the speed of automobiles, the implications of aerodynamic studies are vast. Recognizing these principles ensures that professionals can design and innovate effectively in their respective fields.
Definition of Aerodynamics
Aerodynamics is defined as the study of the motion of air and the forces acting on objects as they move through that air. The subject encompasses various physical laws and concepts, including pressure, viscosity, and flow dynamics. One key aspect is the analysis of lift and drag forces, which are crucial for understanding how objects can achieve flight or minimize air resistance. The branches of aerodynamics can thus be classified into subfields like subsonic, supersonic, and hypersonic aerodynamics, each addressing different velocity regimes.
Historical Context
The history of aerodynamics is rich and marked by significant milestones. Early studies can be traced back to the works of pioneers like Sir George Cayley in the 18th century, who formulated the concepts of lift and drag. The Wright brothers brought these concepts to fruition in 1903 by successfully achieving controlled flight. Since then, advancements in technology have drastically transformed this field. Innovations such as wind tunnels and computational fluid dynamics (CFD) have propelled our understanding of airflow and its effects on various materials and designs. Today, aerodynamics plays an indispensable role in industries that range from aerospace to automotive, effectively influencing performance, fuel efficiency, and safety.
"The evolution of aerodynamics reflects a blend of art and science, where empirical studies have paved the way for theoretical advancements."
As we explore the fundamental principles, methodologies, and applications of aerodynamics testing in subsequent sections, the relevance of its historical context becomes increasingly evident. Understanding the foundational developments allows us to appreciate contemporary techniques and their transformative impact on modern engineering.
Fundamentals of Aerodynamics
The study of aerodynamics is essential to understand the forces that govern the movement of objects through air. It lays the foundation for numerous engineering applications, from the design of aircraft to the optimization of vehicles. A firm grasp of the fundamentals of aerodynamics aids professionals in making informed decisions and improving performance across various domains.
Learning the basic principles equips researchers with the necessary tools to analyze airflow patterns and predict how different surfaces interact with air. This knowledge is particularly important when addressing challenges related to efficiency and safety in aerospace, automotive, and sports engineering.
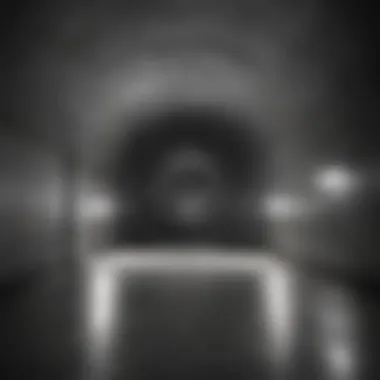
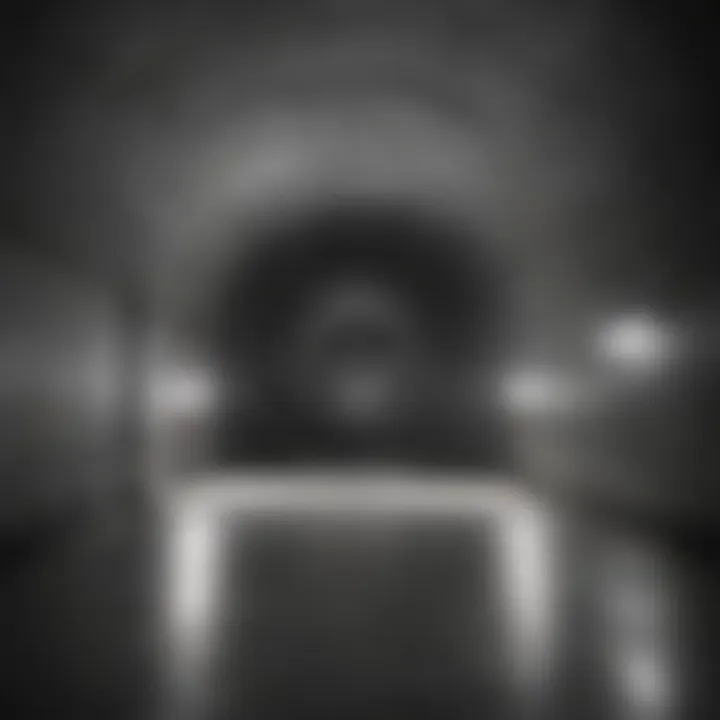
Basic Principles
At the core of aerodynamics lie basic principles that explain how air moves. These principles, such as Bernoulli's principle and the laws of motion, provide insight into various aerodynamic behaviors.
- Bernoulli's Principle: This principle states that an increase in the speed of a fluid occurs simultaneously with a decrease in pressure. It demonstrates how aircraft wings generate lift.
- Newton's Third Law: For every action, there is an equal and opposite reaction. This law explains how thrust is produced by engines and how drag affects motion.
These principles are crucial for understanding how aerodynamic forces interact with different shapes and speeds.
Key Concepts in Fluid Dynamics
Fluid dynamics is the branch of physics that studies the behavior of fluids in motion. In the context of aerodynamics, several key concepts warrant attention.
Viscosity
Viscosity refers to a fluid's resistance to flow. It plays a critical role in the behavior of air when it interacts with surfaces. A fluidβs viscosity affects how it flows over a given shape and influences the drag experienced by that object. For instance, high-viscosity fluids create more resistance, which leads to higher drag forces on objects.
- Key Characteristics: Viscosity is dependent on temperature and pressure, making it essential to consider in various aerodynamics testing scenarios.
- Advantages/Disadvantages: High viscosity can be detrimental for fast-moving objects, as it increases drag. However, understanding viscosity helps engineers tailor designs that minimize resistance in their respective applications.
Flow Patterns
Flow patterns describe the trajectories of fluid particles as they travel around objects. Assessing these patterns is crucial for predicting how an object behaves in the air.
- Key Characteristics: These patterns can be laminar (smooth) or turbulent (chaotic), each influencing drag and lift in distinct ways.
- Advantages/Disadvantages: While laminar flow is desirable for minimizing drag, it can be unstable. Turbulent flow can contribute to increased drag but may also enhance lift. Understanding these dynamics is vital for effective design.
Drag and Lift Forces
Drag and lift forces are fundamental to the study of aerodynamics. Drag force opposes motion, while lift force acts perpendicular to the orientation of the object.
- Key Characteristics: The balance between drag and lift determines the performance and efficiency of vehicles in motion.
- Advantages/Disadvantages: Engineers strive to maximize lift while minimizing drag. Successful manipulation of these forces leads to improved fuel efficiency and overall performance in products like aircraft and vehicles.
Importance of Aerodynamics Testing
Aerodynamics testing plays a crucial role in the development of various industries. Its importance lies in the ability to assess and optimize the performance of vehicles, aircraft, and equipment. Without proper testing, it is difficult to predict how designs will behave under real-world conditions. This could lead to inefficiencies, safety issues, and suboptimal performance. Thus, understanding the significance of aerodynamics testing is essential for both academic researchers and industry practitioners.
Applications in Various Industries
Aerospace
In the aerospace industry, aerodynamic testing is vital for aircraft design. It helps engineers understand how air interacts with different shapes and materials. This understanding leads to the production of safer and more efficient aircraft. A key characteristic of aerospace applications is the necessity for precision; even slight variations in design can lead to significant changes in performance. Additionally, aerospace testing often employs wind tunnels and computational fluid dynamics simulations, making it a robust choice for gathering data. The unique feature of aerospace aerodynamics is its requirement for rigorous standards, which can sometimes be a disadvantage due to high costs involved in testing.
Automotive
Automotive aerodynamics is another important aspect that significantly contributes to vehicle efficiency. It allows manufacturers to minimize drag and improve fuel efficiency. A defining characteristic of automotive testing is its focus on real-world scenarios, such as wind resistance faced by vehicles during operation. This practical approach is beneficial, as it provides insights that can directly translate into performance improvements. However, the unique challenge in automotive aerodynamics lies in balancing aesthetic design with functional efficiency, leading to complexities that might not arise in more utilitarian designs.
Sports Engineering
In sports engineering, aerodynamics testing enhances performance in various sports equipment. From bicycles to swimming suits, understanding airflow can lead to substantial performance gains. A key feature of this application is the drive for competitive advantage, which makes aerodynamics critical. The aspect of innovation in sports is particularly beneficial as materials and shapes can be constantly adapted to enhance athlete performance. However, the ongoing need for new developments sometimes leads to a focus on trends over proven designs, which can be a potential disadvantage in this fast-paced environment.
Benefits of Accurate Testing
Accurate testing in aerodynamics is indispensable due to several benefits it brings. First, it enhances safety by identifying potential failure points before products reach the market. Second, it enables performance optimization, allowing designers to create more efficient and effective products. Lastly, thorough testing provides a competitive edge in the marketplace, as companies that invest in accurate aerodynamics testing are likely to produce superior products.
"Investing in aerodynamics testing is not merely a cost; it's a strategic advantage that determines the success of products in a competitive landscape."
Recognizing these benefits helps stakeholders appreciate the value of aerodynamics testing in driving innovation across multiple domains.
Aerodynamics Testing Methods
Aerodynamics testing methods are critical in understanding how air interacts with objects, particularly in the fields like aerospace and automotive engineering. These methods allow for precise data collection and analysis. They help engineers optimize designs, increase efficiency, and ensure safety. Choosing appropriate testing methods is essential to achieve reliable and informative results.
Wind Tunnels
Wind tunnels are one of the most widely used tools for aerodynamics testing. They control airflow around models and gather data on various forces acting on an object as it moves through air. Their major characteristic is the ability to create controlled testing environments where variables such as speed and airflow direction can be adjusted. This makes wind tunnels a favored choice for researchers and industry professionals alike.
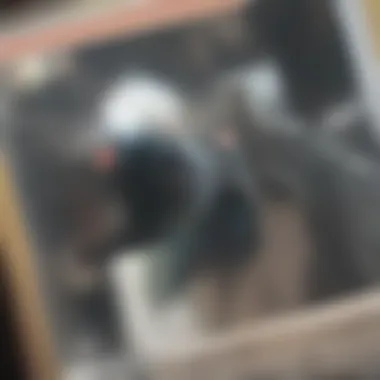
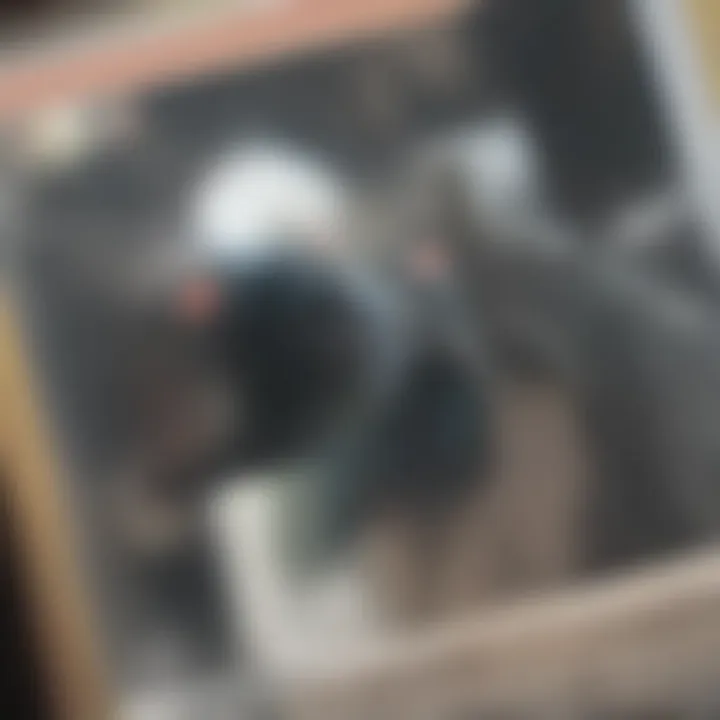
Types of Wind Tunnels
There are various types of wind tunnels, including open-circuit and closed-circuit tunnels.
- Open-Circuit Wind Tunnels: These have air that enters through an inlet and exits into the environment. They are simpler and less costly to build.
- Closed-Circuit Wind Tunnels: These circulate air in a closed loop. They can maintain more steady airflow, which is beneficial for precise measurements.
The main benefit of wind tunnels lies in their ability to simulate real-world conditions. However, one limitation is that they may not fully mimic full-scale flight conditions due to scale effects.
Instrumentation
Instrumentation in wind tunnels refers to the tools and devices used to collect data during tests. This includes pressure taps, balance systems, and flow visualization techniques. Using advanced instruments is important for ensuring data accuracy and reliability.
The key characteristic of instrumentation lies in its capacity to record high-resolution data efficiently. This in turn allows for detailed analysis of air flow patterns and force measurements. However, the complexity of some instruments may require specialized training for proper usage and interpretation of results.
Computational Fluid Dynamics (CFD)
CFD is a powerful method used to simulate and analyze fluid flows around objects. It uses complex algorithms and numerical analysis to solve fluid dynamics equations. Its contribution to aerodynamics testing is significant due to its ability to perform virtual testing before physical models are built, saving both time and cost.
Overview of CFD Techniques
CFD techniques allow for detailed modeling of airflow patterns and interactions with surfaces. They enable engineers to run numerous simulations under various conditions. Moreover, these techniques are widely adopted because they allow for easy adjustments and visualizations of complex aerodynamic phenomena.
However, the accuracy of CFD simulations heavily relies on the quality of underlying models and assumptions made during analysis. This can sometimes lead to discrepancies with real-world results.
Software Tools
There are many software tools available for CFD analysis, such as ANSYS Fluent, OpenFOAM, and COMSOL Multiphysics. These tools provide a range of functionalities, from basic simulations to advanced visualizations and analysis.
The main advantage of these software tools is their versatility. They can cater to various industries and types of problems. However, they usually require substantial computational resources and expertise for optimal use.
Model Testing vs. Full-Scale Testing
Model testing typically involves using scaled-down versions of real objects, while full-scale testing examines the actual size items. Each method has its own set of advantages and challenges.
Model testing is cost-effective and allows for rapid iterations, but it may overlook some scale-related effects that only become apparent at full scale. On the other hand, full-scale tests yield the most accurate results, yet they are often more expensive and logistically challenging.
Understanding the differences and applications of these two testing methods is essential for aerodynamics research and industry practices. Each has its place depending on the specific requirements of a project.
Data Analysis and Interpretation
Data analysis and interpretation play a critical role in aerodynamics testing. After conducting tests, collecting data is only the first step. Interpreting this data accurately determines the insights and knowledge gained from the entire testing process. Effective data analysis enables engineers and researchers to validate designs and optimize performance across various applications.
Collecting Aerodynamic Data
Collecting aerodynamic data is foundational to the process of understanding air movement and its interaction with objects. There are various methods for collecting data, including physical tests conducted in wind tunnels and computational fluid dynamics simulations. Each method offers distinct advantages.
- Wind Tunnel Testing: In this method, models are exposed to controlled airflow. This provides real-time data on forces like drag, lift, and other interactions with the airflow, giving precise measurements.
- CFD Simulations: Computational fluid dynamics allows analysts to visualize airflow in a virtual environment. This enables the evaluation of complex geometries without the need for physical models, facilitating the analysis of scenarios that would be challenging or impossible to test physically.
Analyzing Test Results
After data collection, the next step involves analyzing test results. This analysis is vital to draw conclusions from the data and make recommendations.
Statistical Methods
Statistical methods serve as a bedrock for interpreting results in a meaningful way. By applying robust statistical tools, researchers can ascertain patterns and correlations within the data. A key characteristic of statistical methods is their ability to provide objective, quantifiable insights, which is crucial for decision-making in engineering contexts.
The benefit of using statistical methods lies in their versatility; they accommodate various data types and can help in identifying trends or anomalies. However, there can be limitations, particularly if sample sizes are too small or if data is skewed.
"Statistical methods, when applied correctly, can substantiate hypotheses and guide future investigations."
Visualization Techniques
Visualization techniques enhance the interpretation of aerodynamic data by offering graphical representations of complex numerical data. This method transforms raw numbers into insightful visuals that can facilitate understanding and communication of findings. A key characteristic of visualization techniques is their power to simplify complex datasets, making them accessible to a wider audience.
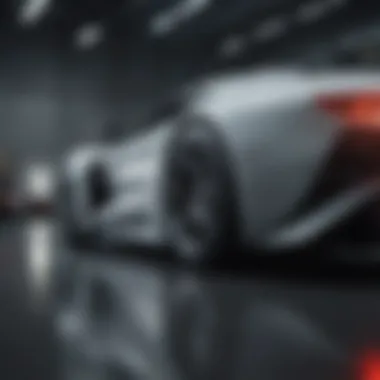
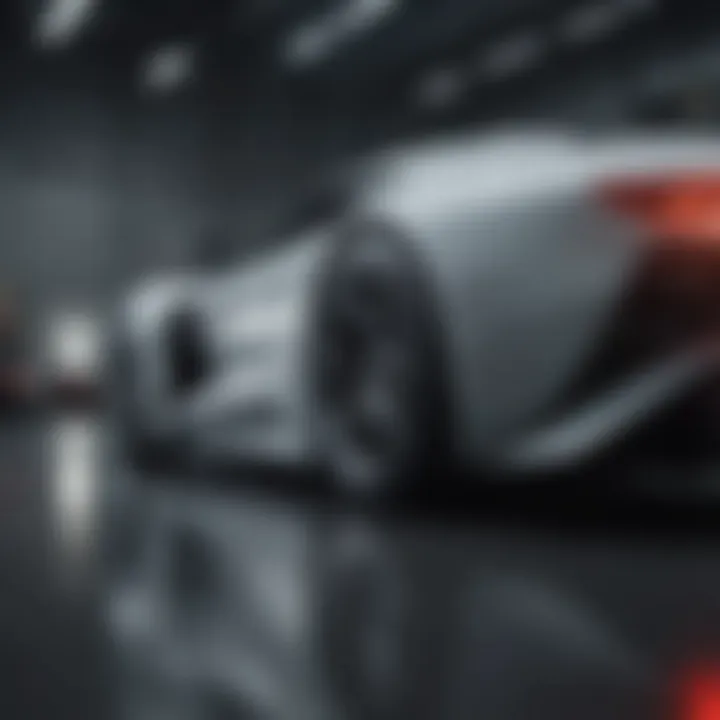
Among the advantages, visualizations can highlight trends that may not be apparent through raw data analysis alone. However, reliance on visuals can sometimes mislead if they are not accurately constructed or if they prioritize aesthetics over clarity.
Utilizing both statistical methods and visualization techniques in concert provides a comprehensive approach to understanding aerodynamic phenomena. Together, they enable engineers and researchers to make informed decisions that enhance design and performance.
Case Studies in Aerodynamics Testing
Case studies in aerodynamics testing provide concrete examples that illustrate the practical applications of theoretical principles. They showcase how the testing methods discussed in earlier sections are employed in real-world contexts. This rich narrative of experiences enables engineers and researchers to learn from past successes and failures, facilitating the drive towards innovation and efficiency. By examining specific cases, stakeholders can glean insights into the nuances and complexities of aerodynamic performance across various fields.
Aerospace Applications
The aerospace industry is perhaps the most prominent domain where aerodynamics testing plays a pivotal role. Various case studies highlight significant advancements in aircraft design and performance through meticulous testing methodologies. For instance, the development of the Boeing 787 Dreamliner exemplifies how aerodynamic testing can lead to substantial improvements in fuel efficiency and overall performance.
Aerodynamic testing on models in wind tunnels facilitated the optimization of wing shapes, which contributes to a decrease in drag. This case illustrates that precise aerodynamic measurements directly affect operational costs and environmental impact.
Additionally, the work done by NASA on the Mars Rover showcased the necessity of testing in extreme environments. The deployment of computational fluid dynamics simulations helped predict how the vehicle would behave during landing in the thin Martian atmosphere. This ensured not only successful landings but also the overall functionality of the rover post-landfall.
Automotive Enhancements
Similarly, the automotive sector has seen transformative effects due to aerodynamics testing case studies. A notable example is the Tesla Model S, which underwent comprehensive aerodynamic testing to surpass competitors in both efficiency and range. The car's streamlined design resulted from systematic wind tunnel experiments, leading to one of the lowest drag coefficients in its category.
In a recent study conducted by Ford on their electric vehicle line, advanced modeling and testing revealed key enhancements in airflow management. The integration of specific features, such as a modified front grille and rear spoilers, demonstrated a direct correlation between testing and driving stability at high speeds. These improvements highlight the continual importance of aerodynamics testing in pushing the boundaries of automotive engineering.
Improving Sports Equipment
The influence of aerodynamics testing also extends to sports equipment. Take the case of cycling, for instance. Research on the design of bicycles for competitive cycling events shows a rigorous application of aerodynamic principles. Teams employ testing methods to analyze everything from rider posture to equipment design.
The aerodynamic frame design of the Pinarello Dogma F12 bicycle is a prime example where extensive testing resulted in critical improvements. By using wind tunnel analysis, engineers were able to create a bike that minimizes drag while maximizing speed. Moreover, individual rider testing provided insights that led to tailored adjustments, significantly enhancing performance.
In swimming, the adoption of new swimsuit technologies reflects similar outcomes from aerodynamics testing. Swimsuits designed with advanced materials reduce water resistance, giving athletes a competitive edge. Case studies of Olympic swimmers illustrate the direct correlation between innovative design, tested aerodynamics, and improved lap times.
Ultimately, these case studies across aerospace, automotive, and sports demonstrate that aerodynamics testing is not just about achieving theoretical understanding but rather a continuous journey of innovation and enhancement.
Challenges in Aerodynamics Testing
Aerodynamics testing serves as a pivotal element in various fields, but it does not come without its set of challenges. Understanding these challenges is essential for advancing testing methodologies and improving practical applications. As industries evolve, so does the complexity of systems that require thorough aerodynamic analysis. Thus, recognizing the limitations and potential future avenues of this testing can inform researchers and practitioners alike in achieving better outcomes.
Limitations of Current Techniques
Despite advancements, current aerodynamics testing methods encounter multiple limitations.
- Cost Considerations: High operational costs of wind tunnel facilities and comprehensive Computational Fluid Dynamics (CFD) resources can restrict usage. Organizations need significant funding to access state-of-the-art testing environments.
- Scale Representation: Testing conducted on models may not always accurately translate to full-scale applications. Scaled tests can introduce discrepancies due to non-linear scaling effects, which affect accuracy.
- Environmental Factors: Tests performed in controlled conditions often overlook the influence of external environments. Factors such as temperature, humidity, and atmospheric pressure can lead to variations that skew results.
- Technology Integration: Existing methods may struggle to fully integrate new technologies, limiting their applicability. As innovations emerge, existing frameworks might not accommodate their unique aerodynamic characteristics.
"The effectiveness of aerodynamics testing is only as strong as its ability to simulate real-world conditions and integrate innovative technologies seamlessly."
These limitations necessitate ongoing assessments to enhance both the reliability and practicality of aerodynamics testing, ensuring validity in results that inform design and safety measures appropriately.
Future Directions for Research
Addressing identified limitations opens avenues for future research within aerodynamics testing. Some promising directions include:
- Enhanced Simulation Techniques: The transition towards more sophisticated CFD techniques that improve simulation accuracy remains vital. By utilizing machine learning and neural networks, researchers can refine their simulations, enhancing predictive capabilities.
- Hybrid Testing Approaches: Combining wind tunnel tests with CFD may yield more comprehensive data. This hybrid method can take advantage of strengths from both worlds, allowing for better validation of models.
- Focus on Real-World Testing: There is value in moving testing beyond laboratory conditions. Real-world experiments that integrate external factors could provide a more accurate reflection of aerodynamic performance.
- Sustainability Initiatives: Future research could address environmentally sustainable practices in testing methodologies. This includes energy-efficient designs for wind tunnels, and adopting alternative resources in simulations, aligning aerodynamics testing with broader environmental goals.
Ending
The conclusion of this article serves as an essential component that encapsulates the understanding of aerodynamics testing. It synthesizes the key findings and emphasizes the significance of these insights across various domains. In a realm where precision and performance are paramount, recognizing the implications of findings from aerodynamics testing is crucial. Key considerations include the impact of advanced testing techniques on design optimization, as well as the relevance of accurate data in real-world applications.
Summary of Key Points
The primary points detailed in this article highlight the foundational principles of aerodynamics and the significance of testing. To summarize:
- Fundamental principles: Understanding the basic concepts of aerodynamics includes the laws governing fluid dynamics, drag and lift forces, and the importance of airflow behavior.
- Testing methodologies: Various techniques such as wind tunnel testing, computational fluid dynamics, and full-scale evaluations are discussed. Each method has unique advantages, contributing to effective aerodynamic analysis.
- Real-world applications: The article provides examples of how aerospace, automotive, and sports engineering sectors benefit from rigorous aerodynamic testing. Enhanced performance and safety are direct outcomes of these practices.
- Challenges and future directions: While current methods face limitations, identifying these challenges paves the way for innovations in aerodynamics testing practices and tools.
Implications for Future Work
The findings from aerodynamics testing extend beyond immediate applications. There are several future directions that can be explored:
- Technological advancements: Emerging technologies, such as machine learning, can refine aerodynamic simulations, leading to more accurate predictions in fluid dynamics.
- Environmental considerations: Research into energy-efficient designs through aerodynamic testing will be critical. This includes addressing sustainability goals in various industries.
- Cross-disciplinary approaches: Collaborations among researchers in different fields can enhance development in aerodynamic testing. Insights from materials science, structural engineering, and environmental science can create more comprehensive testing scenarios.
The future of aerodynamics testing is not limited to just enhancing performance but also includes embracing environmental responsibilities and interdisciplinary innovation. As industries evolve, the principles discussed in this article will continue to guide researchers and practitioners in the quest for optimized designs and improved safety.