Understanding Biased Amplifiers: Theory and Application
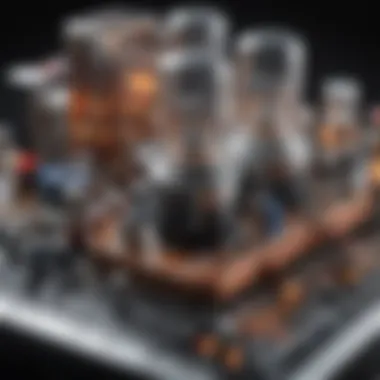
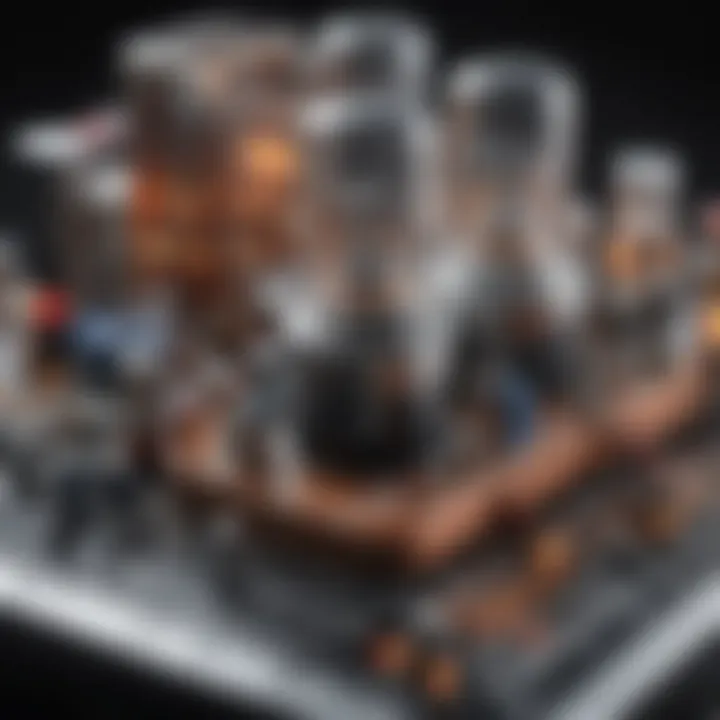
Intro
In modern electronics, understanding biased amplifiers is essential. Their importance cannot be understated, as they play a vital role in various fields such as audio engineering and signal processing. This section will introduce key concepts that are crucial for comprehending the workings of biased amplifiers.
Key Concepts and Terminology
Definition of Key Terms
Biased amplifiers are electronic devices designed to take an input signal and amplify it without altering the original signal's characteristics. They operate in a designated region of the transistor's characteristics to ensure linearity and efficiency.
- Biasing: The process of providing a proper voltage and current to an amplifier's components.
- Linearity: The ability of an amplifier to produce an output signal that is directly proportional to the input.
- Efficiency: A measure of how well an amplifier converts DC power into amplified output.
Concepts Explored in the Article
The article will discuss various biasing techniques and their implications for amplifier performance. Different methods, such as fixed bias, self-bias, and voltage divider bias, will be highlighted.
Also, temperature and load effects on amplifier performance will be examined. Real-world applications of biased amplifiers will be analyzed, showcasing their role in audio devices like microphones and speakers, as well as in other electronic applications.
Findings and Discussion
Main Findings
One significant finding is that the choice of biasing technique can greatly influence an amplifier's linearity and efficiency. Understanding how different factors, such as temperature fluctuations, affect performance is crucial for practical applications.
Potential Areas for Future Research
Future research could explore advancements in biasing methods, particularly in improving efficiency for low-power devices. Exploration into adaptive biasing techniques could also yield substantial benefits in various electronic applications.
"Biasing is not merely a technical requirement but a crucial element that governs the amplifier's operational capacity."
In summary, proficient knowledge of biased amplifiers contributes significantly to advancements in electronic amplification technology. It aids engineers and researchers in developing high-performance systems that meet increasing demands for audio fidelity and signal integrity.
Through this groundwork, a deeper understanding of these devices can foster innovations in future engineering designs.
Foreword to Biased Amplifiers
Biased amplifiers play a critical role in the field of electronics, serving as key components in amplifying signals while maintaining the desired performance characteristics. Their importance lies in a few fundamental elements that shape both their design and application. To start with, biasing helps to establish the operating point of an amplifier, which is essential for achieving linearity and efficiency in signal processing. A properly biased amplifier ensures that the output signal reflects the intended input without significant distortion, thereby enhancing the overall quality of the amplification process.
Another significant aspect is how biased amplifiers are employed across various sectors, including communications, audio engineering, and instrumentation. Each field relies heavily on these amplifiers to ensure proper signal amplification under different conditions, making understanding their design and application crucial for engineers and technologists.
Furthermore, the relationship between biasing techniques and performance parameters such as gain and bandwidth is undeniable. Without adequate bias, amplifiers may exhibit poor performance, leading to challenges in applications that demand high fidelity and reliability.
In summary, the significance of biased amplifiers extends beyond mere signal amplification; it encompasses the optimization of performance metrics and the assurance of high-quality output across multiple technological applications.
Definition and Importance
A biased amplifier is defined as a circuit that utilizes biasing techniques to achieve a stable operating point. This operating point is crucial for linear operation, which means that the amplifier can faithfully reproduce variations in the input signal at its output. The importance of biased amplifiers is multifaceted. Firstly, they maintain signal integrity, allowing for clearer and more accurate reproduction of the input signal. Secondly, the incorporation of appropriate biasing ensures the amplifier works efficiently within its specified parameters, thus preventing issues such as distortion and thermal runaway.
Moreover, in contemporary digital systems relying on analog components, the role of biased amplifiers becomes even more profound. Their ability to handle varying input levels while maintaining consistent output is key to ensuring the performance and reliability of various electronic devices.
Historical Context
The evolution of biased amplifiers traces back to the early days of electronics, where the need for reliable signal amplification became evident. As devices became more complex, the limitations of early amplifiers prompted engineers to explore biasing techniques. During the mid-twentieth century, advancements in semiconductor technology led to the introduction of transistor-based amplifiers, which significantly improved amplification efficiency and performance.
In the 1960s and 1970s, the emergence of integrated circuits heralded a new era in amplifier design. This period saw the refinement of biasing techniques, which facilitated better performance in smaller packages. The advancements made during this time laid the groundwork for today’s sophisticated biased amplifiers used in everything from personal audio devices to high-power broadcast systems.
As technology continues to evolve, the principles of biasing and amplification remain fundamental. The historical context not only underscores the importance of biased amplifiers but also highlights the ongoing need for innovation and optimization in amplifier technology.
Fundamental Principles of Amplifiers
The study of biased amplifiers rests on solid ground marked by the fundamental principles of amplification. These principles provide critical knowledge for understanding how amplifiers function, which is essential for students and professionals alike. In amplifier design and application, it’s crucial to grasp the basic concepts that guide operation, performance, and overall design decisions.
Basic Amplifier Types
Amplifiers can be categorized into various types based on their configurations and functionalities. The most common types include:
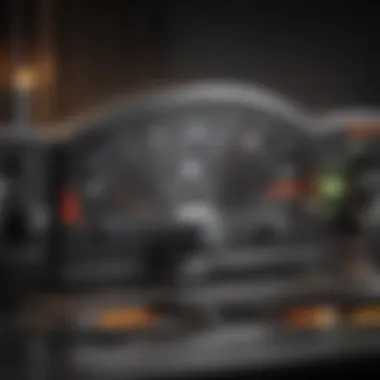
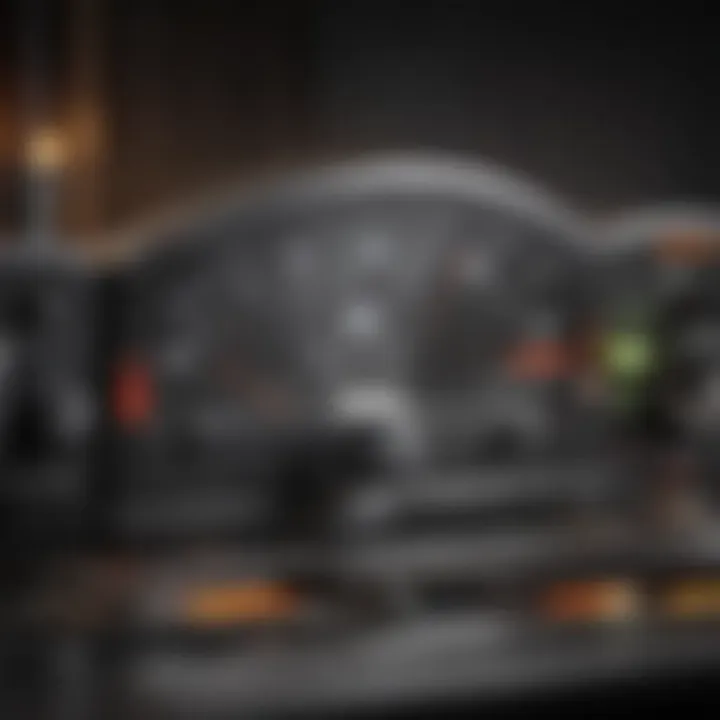
- Common Emitter Amplifiers: These offer high gain and are widely used in voltage amplification. They invert the phase of the input signal, making them suitable for many applications.
- Common Collector Amplifiers: Known as emitter followers, they provide high input impedance and low output impedance. This makes them excellent for impedance matching.
- Common Base Amplifiers: These are less common but provide excellent high-frequency performance. They feature low input impedance, making them suited for RF applications.
Each of these types serves distinct purposes and can significantly affect the performance of a system. Understanding their configurations helps in selecting the right amplifier for specific needs.
Gain and Bandwidth Considerations
Gain and bandwidth are two interrelated fundamental parameters that determine the performance of an amplifier. The gain is a measure of how much an amplifier increases the strength of a signal. It is usually expressed in decibels (dB) and calculated by the ratio of output voltage to input voltage. On the other hand, bandwidth refers to the range of frequencies over which an amplifier operates effectively.
Several points to consider include:
- Trade-off between Gain and Bandwidth: Increasing the gain often results in a narrower bandwidth. This is a direct result of the amplifier's design and is crucial for applications that require specific frequency responses.
- Unified Gain-Bandwidth Product: This concept helps in understanding how gain and bandwidth interact. For most amplifiers, the product of gain and bandwidth remains constant. Designers use this relationship to optimize circuit performance.
- Application-Specific Needs: Depending on the requirements, such as in audio equipment or communication devices, different gain and bandwidth settings may be essential.
Understanding these fundamental principles allows engineers to make informed decisions when designing biased amplifiers, ensuring they meet the required specifications of efficiency and performance. This knowledge also aids in troubleshooting and enhancing existing systems.
"In amplifier design, intuition and fundamental principles guide technical decisions that have real-world impacts."
Overall, a solid comprehension of these aspects forms the backbone of effective amplifier usage, whether in research, education, or professional practice.
Biasing Techniques Overview
Biasing techniques play a crucial role in the performance of amplifiers. Proper biasing ensures that an amplifier functions effectively within its intended parameters, maintaining linearity and minimizing distortion. In biasing, the objective is to establish a stable operating point for the active component, primarily a transistor, to provide consistent operation over varying conditions.
Understanding biasing techniques is essential for several reasons:
- Performance Reliability: Correct biasing provides reliable performance under different environmental conditions.
- Linear Operation: It helps in maintaining the linearity of the amplifier, which is key for accurate signal amplification.
- Distortion Management: Proper biasing can significantly reduce distortion, ensuring signal integrity.
In this section, we will explore two main approaches to biasing: DC biasing and AC biasing, as well as the methodologies behind active and passive biasing methods. Each method has its advantages and challenges, impacting amplifier design and application.
and AC Biasing Explained
DC biasing refers to the process of setting the DC operating point of an amplifier. This is done by applying a DC voltage to the transistor's base (for bipolar junction transistors) or gate (for field-effect transistors). The DC bias point determines how the transistor will behave with incoming signals.
It is important to understand that the biasing affects both quiescent current and collector-emitter voltage in BJTs or drain-source voltage in FETs. These parameters critically influence the amplifier's gain, input, and output impedances. Key points regarding DC biasing include:
- Quiescent Point (Q-Point): The DC biasing configuration establishes the Q-point, ensuring the transistor operates in the active region.
- Bias Stability: Variations in temperature can affect the operating point, so stable designs are necessary.
- Impact on Signal Amplification: Proper DC biasing directly affects how well the amplifier can handle AC signals without distortion.
On the other hand, AC biasing focuses on how an amplifier can respond to varying input signal conditions. Unlike DC biasing, which sets the base or gate level, AC biasing considers the fluctuations and behavior of the signal. The main aspects include:
- Signal Swing: AC coupling through capacitors allows for better handling of input signals without shift in DC operating point.
- Amplification Efficiency: Effective AC biasing can enhance the efficiency of signal amplification, especially in scenarios involving variable signals.
Active and Passive Biasing Methods
Biasing methods can be categorized into active and passive methods, each having its own strengths and applications.
- Active Biasing: This method uses active components like operational amplifiers or transistor amplifiers to set and maintain the biasing point. Active biasing systems can provide stability against fluctuations in temperature and power supply variations. Some benefits of active biasing include:
- Passive Biasing: In contrast, passive biasing employs resistors and passive components to set the bias point. While simpler and often less expensive, passive biasing can be prone to variations, leading to less stability. Key points include:
- Better stability and less drift over time.
- Enhanced performance in varying conditions.
- Simplicity in design and implementation.
- More straightforward maintenance, yet can show variability in performance as conditions change.
Both methods have their unique considerations based on application requirements. The choice between active and passive biasing comes down to design goals, with active biasing often preferred for critical and high-performance applications.
Proper biasing is integral to amplifier design, impacting reliability, linearity, and overall efficiency, making it a pivotal aspect of modern electronic systems.
The Role of Components in Biasing
The efficacy of biased amplifiers rests not just in their design and architecture but significantly in the individual components employed within them. Each element plays a crucial role in shaping the performance and overall characteristics of the amplifier. Understanding the contributions of these components enhances one's grasp of how amplifiers function and the finer points of their operation.
Transistor Characteristics
Transistors are the core active components in most amplifiers, providing the necessary gain to the input signals. Their characteristics determine how well they can amplify a given signal under various conditions. Key parameters to consider include the current gain (h_FE), cutoff frequencies, and thermal stability.
- Current Gain (h_FE): This indicates how much the input current is amplified at the output. A higher h_FE means more efficient amplification, crucial in applications where signal integrity is essential.
- Cutoff Frequency: This represents the frequency at which the gain begins to drop. It's vital, especially when the amplifier is used in high-frequency applications. Ensuring that the transistors maintain performance within the desired bandwidth is a critical factor in design decisions.
- Thermal Stability: Transistors are sensitive to temperature changes. Variations can cause shifts in gain and lead to nonlinear performance or even thermal runaway conditions. A well-designed biasing circuit takes into account these variations, ensuring the amplifier operates effectively across different temperatures.
Resistors and Capacitors Impact
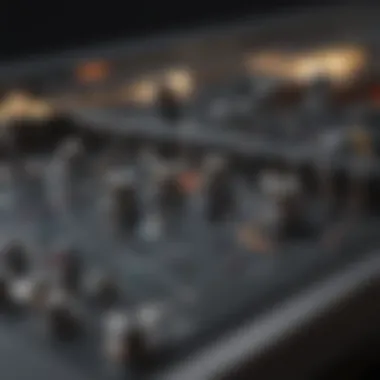
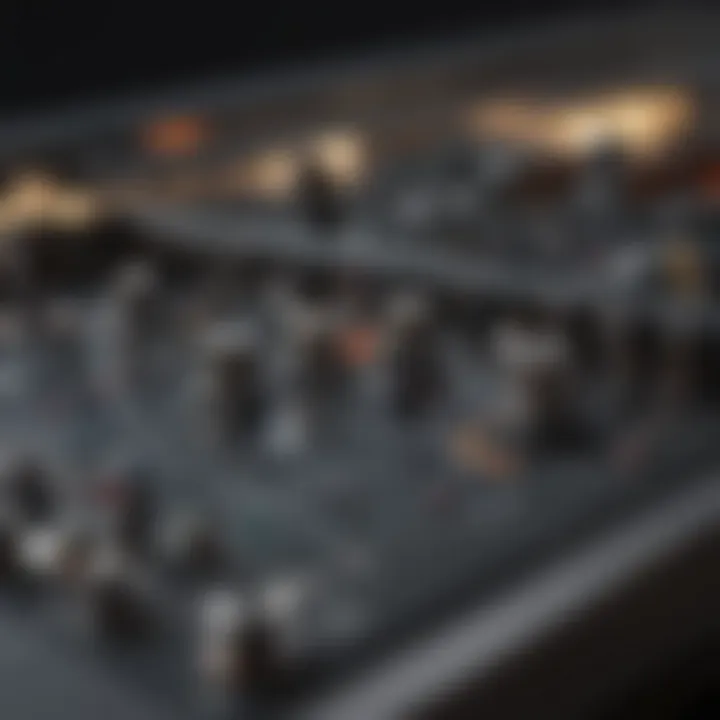
The passive components, resistors and capacitors, are equally essential in defining the behavior of biased amplifiers. They control current flow, shape frequency response, and filter noise, all contributing to the overall performance of the amplifier.
- Resistors: These elements set the biasing point of the transistor, impacting the idle current and ensuring that it operates efficiently in the desired region. They also influence the input and output impedance.
- Capacitors: Used for coupling and bypassing, capacitors ensure that signals pass through without distortion while eliminating unwanted noise. They can impact the bandwidth and frequency response of the amplifier, making their selection critical in design.
In summary, the careful selection and understanding of each component's role allow engineers and designers to optimize biasing techniques for specific applications. Whether working on audio systems or more technical signal processing, recognizing how transistors, resistors, and capacitors interact provides a pathway to achieve design goals effectively.
"The choice of individual components can significantly influence the biasing stability and overall performance of an amplifier."
Deep insight into the roles of these components reveals the underlying complexities of amplifier design. It guides improvements in performance and reductions in distortion, enabling various real-world applications.
Temperature Effects on Biased Amplifiers
Temperature plays a crucial role in the performance and reliability of biased amplifiers. Understanding the effects of temperature on amplifier operation is vital for both design and application. As temperature varies, so do the electrical parameters of components, which can alter an amplifier's behavior. This section addresses two specific elements that are key to grasping the temperature dynamics in amplifiers: the thermal runaway phenomenon and the compensation techniques employed to mitigate these issues.
Thermal Runaway Phenomenon
Thermal runaway is a condition where an increase in temperature causes an increase in current, which in turn leads to a further rise in temperature. This creates a positive feedback loop that can lead to damage or failure of the amplifier.
The primary cause of thermal runaway in biased amplifiers is the behavior of bipolarjunction transistors, or BJTs. As the temperature inside the transistor increases, the base-emitter junction's forward voltage drop decreases. Consequently, more current flows through the transistor. If the biasing is not carefully controlled, this can lead to an uncontrollable rise in current and thus temperature.
"In a poorly designed amplifier, thermal runaway can occur unnoticed until irreversible damage has been done."
To avoid this phenomenon, engineers must account for temperature variations during the design phase. Properly selecting biasing resistors can help maintain a stable operating point across a range of temperatures. Furthermore, choosing components with suitable thermal characteristics is essential to reduce the risk of thermal runaway.
Compensation Techniques
Compensation techniques are strategies employed to counteract the effects of temperature fluctuations on biased amplifiers. These techniques aim to stabilize the operating point of the amplifier, ensuring reliable performance across varying thermal conditions.
- Negative Feedback: One common method is to implement negative feedback within the amplifier circuit. This feedback helps to reduce the gain as temperature rises, thus limiting current flow and preventing thermal runaway.
- Temperature Coefficient Matching: Engineers can also design circuits using components that have similar thermal characteristics. This way, variations in temperature can affect them uniformly, helping maintain stable amplifier operation.
- Thermal Sensors: Some designs include thermal sensors that monitor the temperature of critical components. If the temperature exceeds a predefined limit, the amplifier can adjust its gain or power to avoid overheating.
- Active Cooling Methods: In high-power applications, active cooling methods such as heatsinks or fans can be utilized. These solutions can dissipate heat away from sensitive components, maintaining optimal operating conditions.
By employing these compensation techniques, designers can ensure that biased amplifiers remain functional even in the face of temperature variations. Understanding these dynamics is essential for building reliable, long-lasting electronic systems.
Load Considerations in Amplifier Design
The design of amplifiers is heavily influenced by load considerations. Load affects how well an amplifier performs and interacts within an electronic system. When designing biased amplifiers, understanding the load is essential. Designers must consider the impedance of the load to ensure the amplifier operates effectively. A mismatch between the amplifier and the load can lead to undesired consequences such as distortion and reduced efficiency.
Understanding Load Impedance
Load impedance refers to the opposition that a load presents to the flow of electrical current. It can vary greatly between different applications. In audio applications, for instance, load impedance is often set at specific values, such as 4 ohms or 8 ohms for speakers. This impedance is critical because it determines how much power can be transferred from the amplifier to the load. A correct match ensures that the maximum power transfer occurs, which is governed by the maximum power transfer theorem. Load impedance can be measured using various methods, including using an LCR meter or by analyzing the circuit behavior.
Important Note: Proper load impedance matching is necessary to minimize signal reflection and loss in electronic systems.
The reflected signal can lead to inefficiencies. Designers must ensure that the amplifier output matches the load for optimal performance. Furthermore, understanding the frequency response of both the amplifier and the load can also provide insights into how they interact across different frequencies, which is paramount in achieving desired audio qualities.
Impact on Amplifier Efficiency
The efficiency of an amplifier is significantly impacted by the load connected to it. Efficiency is defined as the ratio of output power to input power. A well-matched load can enhance this efficiency, leading to higher overall performance. If an amplifier is overloaded, it can lead to overheating and distortion, which can downscale the performance drastically. Conversely, an underloaded amplifier might not utilize its capacity effectively, leading to wasted power.
There are a few specific elements to consider regarding the impact of load on amplifier efficiency:
- Load Characteristics: The nature of the load, whether resistive or reactive, plays a role in determining how efficiently power is used. Resistive loads absorb power effectively, while reactive loads may store energy without dissipating it.
- Output Power: Output power should be matched with the load capabilities. Overdriving an amplifier can result in clipping, limiting the efficiency.
- Heat Dissipation: Increased load can also lead the amplifier to dissipate more heat. Therefore, cooling methods and materials should be factored into the design to maintain efficiency during operation.
Applications of Biased Amplifiers
The applications of biased amplifiers are critical in various fields, particularly in electronics. These amplifiers serve as the backbone for many devices, enhancing signal strength, ensuring clear sound, and improving overall performance. Understanding their applications allows for better designs and implementations that meet specific demands within audio and communication sectors.
In audio engineering, biased amplifiers play a vital role in delivering high-quality sound. They help in maintaining the fidelity of audio signals, enabling accurate reproduction. By carefully selecting biasing methods, engineers can tailor the performance of these amplifiers to meet both signal integrity and tonal quality requirements. This customization can greatly affect the listening experience, making proper biasing essential for audio devices.
In communication systems, the application of biased amplifiers becomes even more significant. These amplifiers are essential for boosting weak signals over long distances. Communications rely on the precision of these amplifiers to ensure that information is transmitted efficiently without degradation. Modern communication equipment, like wireless transmitters and receivers, heavily depend on well-designed biased amplifiers to maintain clarity and reliability.
Overall, the study and understanding of biased amplifier applications contribute to various benefits:
- Improved Signal Quality: Ensures the clarity and integrity of audio and data.
- Enhanced Performance: Tailors devices to operate efficiently within their specific applications.
- Adaptability: Offers diverse uses in different fields, from music to telecommunications.
Integration of biased amplifiers into technology not only leads to practical advancements but also shapes the future of electronic innovations.
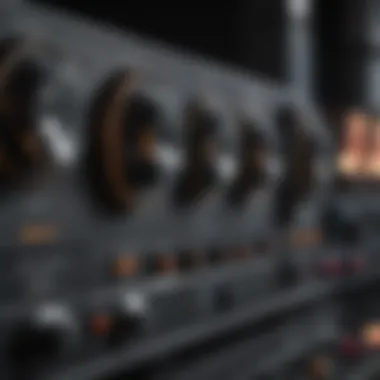
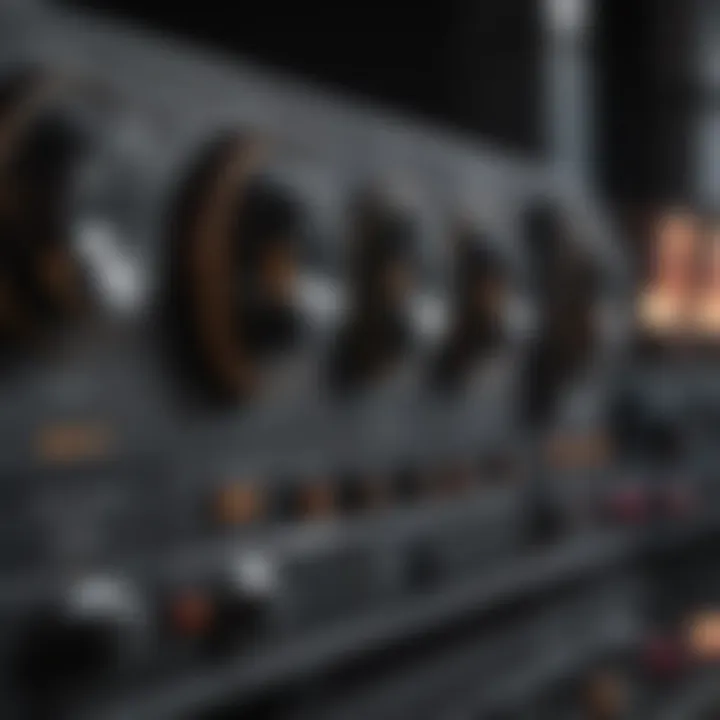
Challenges in Biased Amplifier Design
Biased amplifier design presents several challenges that engineers and developers must address to ensure optimal performance. These challenges can significantly impact the amplifier’s functionality, efficiency, and application suitability. Understanding these hurdles is vital for students, researchers, educators, and industry professionals aiming to grasp the intricacies of amplifier technology.
Distortion and Linearity Issues
Distortion and linearity are two critical factors affecting biased amplifier performance. Distortion refers to any alteration of the signal during amplification, which can degrade output quality. Non-linear amplification can lead to harmonic distortion, where the output signal produces unwanted frequencies. This is especially crucial in audio applications where clarity is paramount.
To mitigate distortion, designing for linearity is essential. Designers often analyze the amplifier’s transfer function to ensure that the output faithfully represents the input signal. Several methods can be adopted to improve linearity:
- Negative feedback: Implementing negative feedback can significantly reduce distortion, allowing for better linearity across different operation levels.
- Bias point adjustment: By strategically positioning the bias point in the active region of the transistor's characteristics, distortion can be minimized.
- Component selection: The choice of transistors and load resistors can influence linearity. High-quality components typically exhibit better linear performance.
Through careful attention to these aspects, engineers can achieve a more linear response in biased amplifiers, thereby enhancing the integrity of the amplified signal.
Power Consumption vs. Performance Trade-offs
In the quest for high-performance biased amplifiers, power consumption remains a significant concern. Designers must balance the need for optimal performance with the electrical efficiency of the device. High-performance amplifiers often demand more energy, leading to increased power consumption and heat generation.
Some critical considerations include:
- Class of operation: Amplifiers operate in various classes (e.g., Class A, Class B, or Class D), each possessing distinct power efficiency profiles. Class A amplifiers provide superior linearity at the cost of higher power usage, while Class D amplifiers are more efficient but can introduce distortion.
- Load matching: Properly matching the load can lead to better efficiency. However, this can be challenging when multiple devices operate simultaneously, as load dynamics can change.
- Dynamic range: A broad dynamic range in an amplifier can enhance overall performance but often increases the complexity of biasing circuits, resulting in higher power demand.
Making informed trade-offs is crucial. Engineers must analyze application requirements and performance expectations to decide the best amplifier class and design parameters. Achieving the right balance ultimately defines the amplifier's suitability for specific tasks.
Future Trends in Amplifier Technology
Future trends in amplifier technology influence numerous industries, from audio engineering to telecommunications. As the demand increases for higher efficiency, better integration with digital systems, and new material usage, the relevance of understanding these trends can’t be overstated. The evolution of biased amplifiers is closely tied to these developments. Those engaged in electronics must consider how advancements in technology will shape the design and functionality of amplifiers in the coming years.
Integration with Digital Systems
Amplifiers are increasingly integrated with digital systems. This convergence brings advantages. Among them is improved control over amplification characteristics. Digital signal processing allows for more precise and adaptable biasing of amplifiers, tailoring responses to specific requirements. For instance, when integrating with digital audio systems, amplifiers can process and enhance audio signals, resulting in superior sound quality.
The synergy between digital and analog technologies manifests in several aspects:
- Increased Efficiency: Integration reduces power consumption and heat generation, essential in portable and battery-operated devices.
- Enhanced Functionality: Digital control opens doors to new functionalities. Programmable amplifiers can adapt features like gain, bandwidth, and distortion characteristics in real time.
- Improved Calibration: Digital systems can assist in calibrating amplifiers for optimal performance, adjusting for varying conditions without manual intervention.
This integration is not without challenges. Developers must navigate complexities associated with analog-digital compatibility and signal fidelity. Nevertheless, the shift toward digital methods marks a significant advancement in amplifier technology.
Emerging Materials and Methods
The exploration of emerging materials and methods is paving the way for revolutionary changes in amplifier designs. Traditional materials are being replaced with advanced compounds that enhance performance.
Graphene is one such material that has garnered attention for its impressive electrical properties. Its utilization in amplifier components can lead to improved efficiency and reduced noise, presenting opportunities for achieving higher fidelity in audio signals. Other materials, such as carbon nanotubes and wide bandgap semiconductors, are also becoming prominent in amplifier technology due to their enhanced performance capabilities at elevated temperatures and frequencies.
Additionally, innovative methods of fabrication are on the rise. Techniques such as 3D printing enable streamlined production processes of amplifier components. This not only reduces manufacturing costs but also allows for complex geometries that can improve performance. Examples of trending methods include:
- Flexible Electronics: These allow for lightweight and adaptable designs that can be integrated into various applications, especially in wearable technology.
- Hybrid Amplifier Design: By merging different amplifier technologies, engineers can optimize for specific applications like software-defined radios.
"The future of amplifier technology is not just in maintaining analog principles; it involves adapting and evolving to a digital-centric world, ensuring efficiency and fidelity."
Researching these developments is crucial for anyone committed to advancing amplifier technology.
End: The Path Forward
In the world of electronics, understanding biased amplifiers holds pivotal significance. Pointing toward contemporary advancements, this article delineates the intricate balance necessary for optimizing amplifier performance. The essence of biasing is not merely in enhancing gain but in ensuring stability, linearity, and efficiency under varying conditions. By analyzing the various biasing techniques and the roles of components, the reader can appreciate the multipronged challenges faced in real-world applications.
One can't overlook that the evolution of biased amplifiers is intrinsically linked with the progress of electronic technology. The rise of digital systems and emerging materials, such as graphene, beckons a new era in amplifier design. These innovations promise improved performance metrics while addressing the constraints faced by traditional amplifiers. The convergence of analog and digital realms can redefine how we utilize these critical components in various domains.
Summary of Key Insights
Biasing amplifiers plays a crucial role in their functionality. The key insights from this article emphasize:
- Diverse Applications: Biased amplifiers are integral in fields such as audio engineering and signal processing, showcasing their versatility.
- Thermal Management: Understanding thermal runaway and employing compensation techniques are vital in maintaining performance stability.
- Trade-offs: Designers face critical decisions regarding performance versus power consumption, often necessitating a nuanced approach to amplifier design.
"Mastery of biasing techniques is essential for advancing amplifier technology in the electronics landscape."
These insights lay a solid foundation for practitioners and researchers, guiding their efforts in amplifier design and optimization.
Further Research Directions
The field of biased amplifiers stands at the precipice of significant innovation. Future research directions include:
- Integration with Digital Systems: Exploring how traditional biased amplifiers can effectively interface with emerging digital technologies is vital for their evolution.
- Investigation into New Materials: The study of materials like graphene and their application in amplifier design could yield remarkable results in performance enhancement.
- Improved Biasing Techniques: Continued development of biasing methodologies that can adaptively respond to changes in operating conditions is essential for next-generation amplifiers.