Understanding mRNA: Its Definition and Impact
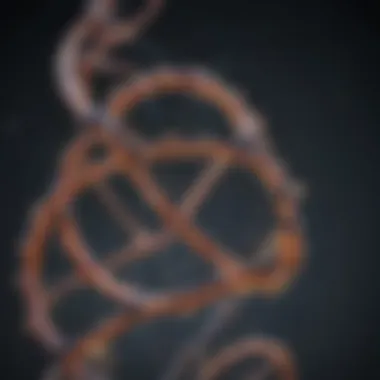
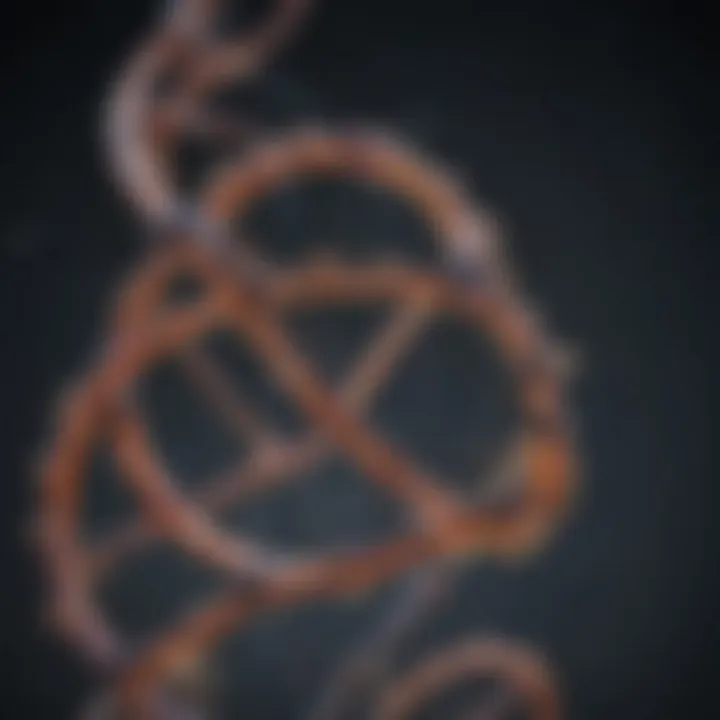
Intro
The exploration of messenger RNA, commonly known as mRNA, stands at the forefront of contemporary molecular biology and medicine. Recent years have revealed significant advancements in the understanding and application of mRNA, particularly in the field of vaccine development. As such, comprehending the functions and implications of mRNA provides essential insights into biological processes and therapeutic innovations.
This article examines not only the definition of mRNA but also its roles in genetic expression and the broader implications for modern medicine. Through a detailed analysis, we will explore how mRNA acts as a template for protein synthesis, its applications in biotechnology, and its transformative potential in disease prevention and treatment.
Understanding these concepts is crucial for students, researchers, educators, and professionals who are keen to engage with the latest developments in molecular biology and medicine.
What is mRNA?
Messenger RNA, commonly known as mRNA, serves a crucial role in cellular biology. Understanding mRNA is essential for grasping how genetic information is converted into functional proteins. As we explore mRNA, we uncover its foundational importance not just in cellular processes but also in modern scientific advancements, particularly in medicine and biotechnology. The following sections will illuminate the definition of mRNA, its structural characteristics, and its implications in both natural and applied contexts.
Defining mRNA
mRNA, or messenger ribonucleic acid, is a type of nucleic acid that encapsulates genetic instructions from DNA. Unlike DNA, which is double-stranded, mRNA is single-stranded and serves as an intermediary molecule. Its primary function is to convey genetic information necessary for synthesizing proteins. This process begins with transcription, where enzymes read a segment of DNA to produce mRNA. The mRNA subsequently transports this information from the nucleus to the cytoplasm, where it guides protein synthesis.
mRNA is also pivotal in understanding how cells respond to various stimuli, adapt to their environments, and differentiate in function. This dynamic nature makes mRNA not only a fundamental aspect of biology but also a focal point in research and therapeutic development.
Basic Structure of mRNA
The structure of mRNA is straightforward yet highly functional. It comprises several key components that contribute to its role in protein synthesis:
- Nucleotide Sequence: mRNA is built from nucleotides, which include adenine (A), uracil (U), cytosine (C), and guanine (G). This sequence determines the specific instructions for protein synthesis.
- 5' Cap: This modified guanine nucleotide is attached to the 5' end of mRNA and protects it from degradation. It also assists in ribosome recognition during translation.
- Poly-A Tail: Located at the 3' end, this stretch of adenine nucleotides enhances mRNA stability and aids in the export of mRNA from the nucleus.
- Open Reading Frame (ORF): This is the critical sequence that encodes the protein. The ORF contains codons, groups of three nucleotides that specify a particular amino acid.
The architecture of mRNA allows precise regulation of gene expression, making it a central element in biotechnological applications and research.
Understanding mRNA's definition and structure sets the foundation for examining its biological role, which we will delve into in the next section.
The Biological Role of mRNA
The role of messenger RNA (mRNA) in biological systems is crucial for many processes that sustain life. This section aims to outline the significance of mRNA in gene expression and protein synthesis, two fundamental biological processes. Understanding how mRNA functions informs both basic scientific research and applied medicine, especially in the context of developing targeted therapies and vaccines.
The biological role of mRNA can be divided into two main processes: transcription and translation. These processes are foundational for how genetic information is converted into functional proteins, which are essential for cellular structures and functions.
Transcription Process
Transcription is the first step in gene expression, where the DNA sequence of a gene is copied to produce mRNA. This process occurs in the nucleus of eukaryotic cells and involves several key steps:
- Initiation: Transcription begins when the enzyme RNA polymerase binds to a specific region of the DNA called the promoter. This binding is crucial for starting the transcription process.
- Elongation: As RNA polymerase moves along the DNA template strand, it synthesizes a complementary mRNA strand. This mRNA strand is built in the 5' to 3' direction, meaning nucleotides are added at the 3' end. The RNA polymerase unwinds the DNA, allowing access to the coding sequence while adding ribonucleotides to the growing mRNA chain.
- Termination: Transcription continues until the polymerase reaches a termination signal in the DNA sequence. At this point, RNA processing begins, which includes the addition of a 5' cap and a poly-A tail. These modifications are essential for mRNA stability, transport, and translation efficiency in the cytoplasm.
"Transcription is the critical first step in translating genetic information into functional products within the cell."
This process ensures that the genetic code contained in the DNA is accurately copied into mRNA, setting the stage for the next essential process in gene expression: translation.
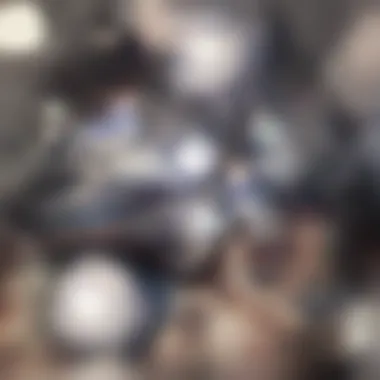
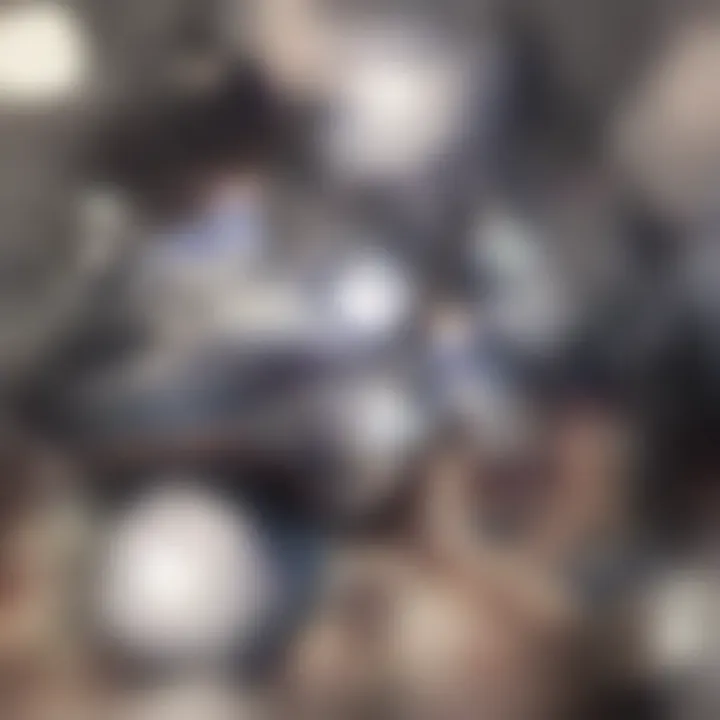
Translation Mechanism
The translation mechanism is where the mRNA produced during transcription is used to synthesize proteins. This process occurs in the cytoplasm and involves ribosomes, transfer RNA (tRNA), and various other factors. Translation can be broken down into three main steps:
- Initiation: The start of translation occurs when the ribosome assembles around the mRNA strand. It identifies the start codon, usually AUG, where synthesis will begin. An initiating tRNA molecule carrying methionine recognizes this codon and binds to the ribosome.
- Elongation: During elongation, tRNA molecules bring specific amino acids to the ribosome, corresponding to each codon on the mRNA strand. As the ribosome moves along the mRNA, amino acids are linked together in a growing polypeptide chain via peptide bonds. This stage is where the actual construction of proteins occurs, translating the genetic code into functional units.
- Termination: Translation ends when the ribosome reaches a stop codon on the mRNA. At this point, the completed polypeptide chain is released, and the ribosome disassembles. The newly synthesized protein then undergoes folding and post-translational modifications to become fully functional, ready to perform its specific role in the cell.
The understanding of the transcription and translation processes highlights mRNA's importance in linking genotype to phenotype, opening doors to biotechnological applications and therapeutic innovations.
mRNA vs DNA
Understanding the distinction between mRNA and DNA is crucial in the realm of molecular biology. Both molecules play pivotal roles in the flow of genetic information within an organism. However, they have fundamentally different structures, functions, and implications in biological systems. This section will dissect their characteristics and explore how they interrelate to influence cellular processes.
Key Differences
mRNA and DNA differ in several key aspects that are essential for their specific roles in the cell. Here are some of the primary differences:
- Structure: DNA is typically double-stranded, featuring a helical structure, while mRNA is single-stranded. This difference gives mRNA a more flexible configuration, allowing it to perform its functions in the cytoplasm more efficiently.
- Nucleotide Composition: DNA contains the nucleotides adenine, cytosine, guanine, and thymine. In contrast, mRNA also contains adenine, cytosine, and guanine but substitutes uracil for thymine. This substitution is significant for the processes of transcription and translation.
- Function: DNA serves as the long-term storage of genetic information, acting as a template for replication. mRNA, on the other hand, is a transient molecule that conveys the genetic code from DNA to the ribosomes for protein synthesis. It serves as a messenger, indicating how the genetic information should be translated into proteins.
- Stability: DNA is chemically more stable than mRNA due to its double-helix structure and absence of reactive hydroxyl groups on the 2'-carbon of the ribose sugar. This stability is crucial, as it ensures the integrity of genetic information over time. Conversely, mRNA is designed to be relatively unstable, which allows it to be quickly synthesized and degraded as needed.
In summary, the differences between mRNA and DNA are not merely a matter of structure; they reflect the distinct roles each molecule plays within the cell.
Functional Relationships
The relationship between mRNA and DNA is foundational for the function of living organisms. Their interaction is central to the process known as the Central Dogma of molecular biology, which describes the flow of genetic information:
"DNA makes RNA makes Protein"
- Transcription: The first step in this process is transcription, where a segment of DNA is copied into mRNA. This occurs in the nucleus of eukaryotic cells, involving RNA polymerase, which synthesizes an mRNA strand complementary to the DNA template.
- Translation: Once mRNA is formed, it travels from the nucleus to the cytoplasm, where ribosomes read the mRNA sequence to synthesize proteins. This is the translation step, where the information carried by the mRNA is converted into functional products.
Thus, mRNA acts as the intermediary that allows DNA's information to be utilized for synthesizing proteins necessary for cellular functions. The successful interaction between mRNA and DNA is vital for maintaining the organism's physiological processes, making it a core concept in both genetics and biotechnology.
The interplay between these two molecules emphasizes the dynamic nature of genetic expression, illustrating how cellular machinery efficiently uses the information encoded within the genetic material.
Applications of mRNA in Biotechnology
The application of mRNA in biotechnology represents a significant leap in various fields, particularly in medicine and therapeutic development. mRNA technology has proven to be transformative, leveraging the naturally occurring molecular messenger for applications that revolutionize how we approach diseases. This section will focus on two critical aspects: using mRNA as a therapeutic agent and its role in vaccine development.
mRNA as a Therapeutic Agent
mRNA has gained attention as a promising therapeutic agent in recent years. Traditional approaches to treatment often involve directly targeting proteins or use of DNA manipulation, but mRNA offers a new paradigm. Using mRNA to instruct cells to produce specific proteins can influence therapeutic options significantly.
The ability of mRNA to elicit a response within cells means it can potentially treat a range of conditions, from rare genetic disorders to cancers. For example, mRNA-based treatments can promote the production of therapeutic proteins that are dysfunctional in certain diseases. This approach is beneficial because it mimics the bodyβs natural processes and can lead to more sustainable and effective treatments.
"mRNA has the potential to enhance targeted therapy by allowing for a more controlled and specific approach."
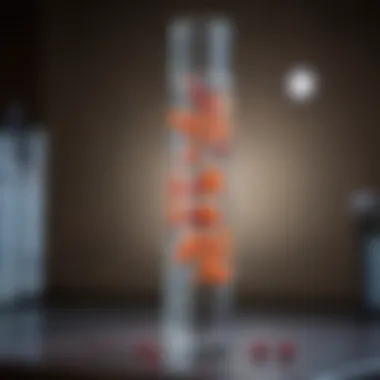
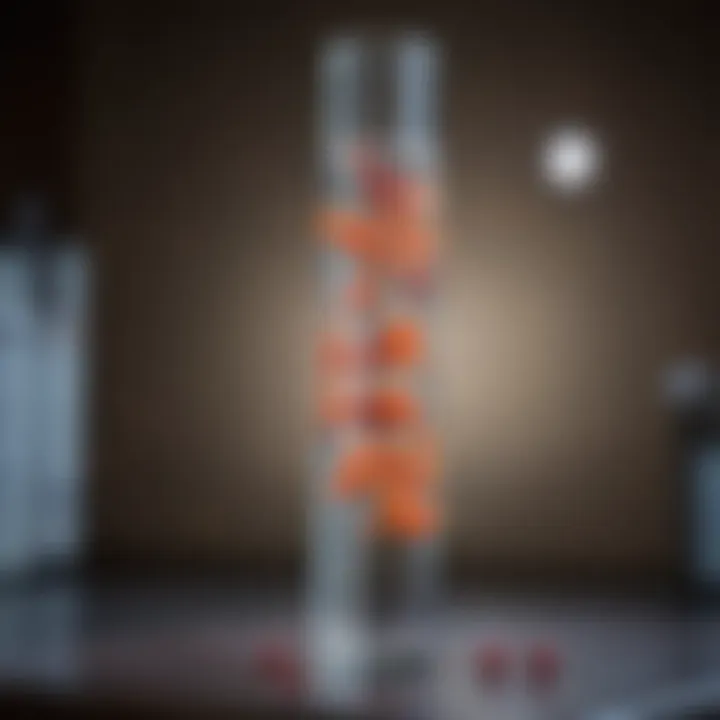
However, the design and delivery mechanisms remain critical challenges in this field. Ensuring that the mRNA reaches the right cells in sufficient quantities is essential. Advances are ongoing to develop lipid nanoparticles and other carriers that can improve the stability and delivery of mRNA, allowing for more effective therapeutic outcomes.
Role in Vaccine Development
The role of mRNA in vaccine development has especially stood out during recent global health challenges. mRNA vaccines, such as those developed by Pfizer-BioNTech and Moderna, have changed the landscape of immunization. Instead of traditional methods that use attenuated or inactivated pathogens, mRNA vaccines instruct cells to produce a harmless piece of a virus, prompting an immune response without the risk associated with exposing the organism to the actual virus.
One of the primary advantages of mRNA vaccines is their speed of development. Once the genetic sequence of a virus is known, mRNA vaccines can be designed and produced rapidly. This technology has been pivotal in the swift response to the COVID-19 pandemic, showcasing the efficacy and adaptability of mRNA in emergency situations.
Moreover, these vaccines can be tailored easily to target different pathogens. As new variants of viruses emerge, modifying mRNA to ensure continued protection could keep vaccination efforts ahead of evolving disease threats. This capability could extend beyond infectious diseases and include potential applications in cancer immunotherapy and other areas.
Recent Advances in mRNA Research
Recent advancements in mRNA research have significantly transformed the landscape of biotechnology and medicine. The exploration into mRNA technology is crucial because it has opened new pathways for understanding and manipulating genetic information. Notably, these advances contribute to substantial improvements in therapeutic strategies and vaccine responses, especially in the wake of global health challenges.
Innovations in mRNA Technology
The field of mRNA technology has seen remarkable innovations that enhance its effectiveness and reliability. Key developments include the refinement of mRNA synthesis methods, resulting in higher yields and purities. Furthermore, researchers have been working on improving the stability of mRNA molecules. Stability is essential because it prolongs the lifespan of these molecules in biological systems, ensuring they perform their functions effectively.
Efforts such as lipid nanoparticle (LNP) encapsulation have gained traction. These nanoparticles protect mRNA molecules from degradation and facilitate their delivery to target cells.
- mRNA Modification: Modified nucleotides are increasingly incorporated to reduce immune reactions and increase translation efficiency.
- Delivery Methods: Alongside LNPs, viral vectors are explored as alternative delivery systems for mRNA.
- Biomanufacturing: New technologies have been developed to produce mRNA on a larger scale, making it more accessible for research and clinical use.
These innovations significantly impact therapeutic applications, making mRNA a promising platform in treating a range of diseases, including cancers and genetic disorders.
Clinical Trials and Results
Clinical trials represent the critical phase where theoretical advancements are put to practical tests. Recent studies have highlighted the potential of mRNA technology in real-world scenarios, particularly in vaccine development for diseases such as COVID-19. The results from these trials have established new benchmarks for speed and efficacy in vaccine production.
Notably:
- The mRNA vaccines developed by Pfizer-BioNTech and Moderna have shown efficacy rates exceeding 90% in preventing symptomatic COVID-19.
- Ongoing trials are exploring mRNA applications beyond infectious diseases, including therapeutic vaccines for cancer.
- The adaptability of mRNA technology allows for rapid modification in response to emerging threats, showcasing its potential in public health.
Given these results, there is a growing optimism about the role of mRNA in future therapeutic strategies. The implications are vast, from rapid vaccine deployment during pandemics to personalized medicine approaches tailored to individual genetic profiles. This area of research remains dynamic and could reshape how we approach health crises in the coming years.
"mRNA technology stands at the forefront of medical science, promising not just to tackle existing diseases but to innovate how we approach them entirely."
Challenges in mRNA Therapeutics
mRNA therapeutics represent a groundbreaking advance in biotechnology and medicine. However, there are significant challenges that must be addressed to realize their full potential. These challenges encompass a range of technical, ethical, and biological factors that influence the development, deployment, and acceptance of mRNA-based therapies.
Stability and Delivery Mechanisms
A primary challenge in mRNA therapeutics is the stability of mRNA molecules. mRNA is inherently unstable. It can degrade rapidly if exposed to environmental factors such as temperature, pH, and enzymatic action. This short lifespan poses problems for storage and transportation.
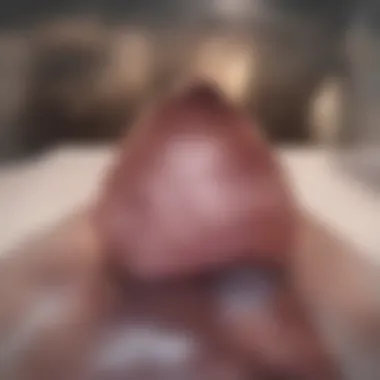
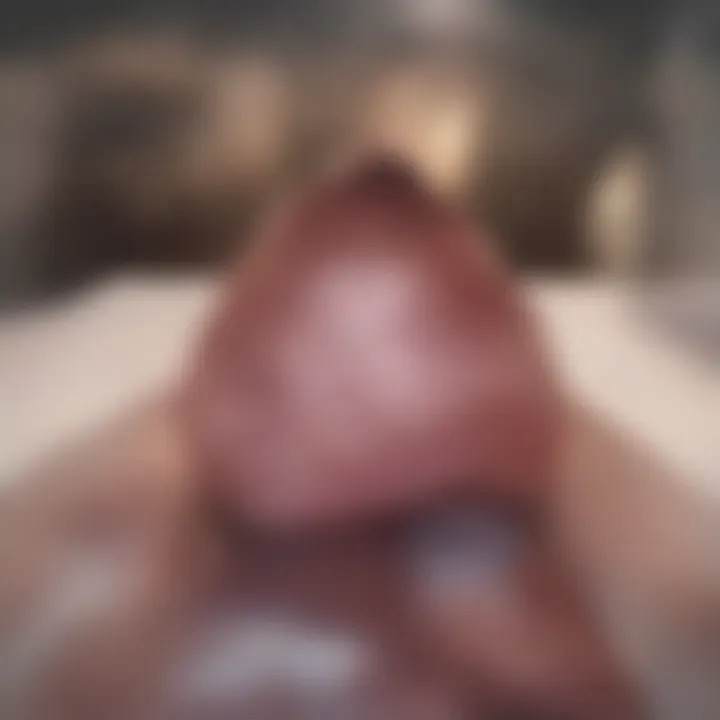
For effective therapeutic use, mRNA must reach its target cells intact. The delivery mechanisms are critical. Various methods are being explored. These include lipid nanoparticles, electroporation, and viral vectors. Lipid nanoparticles, in particular, have gained traction. They protect mRNA from degradation. They also facilitate cellular uptake. However, optimizing these delivery systems continues to require extensive research.
"The efficacy of mRNA therapeutics relies heavily on efficient delivery mechanisms which ensure stability and cellular uptake."
Yet, achieving a balance between efficiency and safety remains a daunting task. Potential toxicity from delivery vehicles can arise. This necessitates careful evaluation to ensure that the benefits outweigh any associated risks.
Ethical Considerations
Ethical concerns in mRNA therapeutics cannot be overlooked. As these treatments advance, they prompt discussions about consent, access, and the potential for misuse. For instance, the rapid development of mRNA vaccines during the COVID-19 pandemic highlighted how urgent public health needs can bypass some typical regulatory processes.
Access to mRNA therapies also raises ethical questions. Equity in availability is a significant concern for researchers and policymakers. Ensuring that these life-saving treatments reach underserved populations is essential for ethical practice in medicine. Moreover, disparities in health resources could exacerbate health inequities if not addressed properly.
The potential for genetic modification using mRNA also invites ethical scrutiny. Gene editing technologies may offer new healing possibilities, but they also pose risks if misused. Discussions surrounding the governance and regulations that guide these technologies are vital.
Future Directions in mRNA Research
The exploration of future directions in mRNA research is critical in the context of ongoing advancements in biotechnology and medicine. As researchers continue to unlock the potential of messenger RNA, various key areas promise not only to enhance our understanding of biological processes but also to offer breakthroughs in therapeutic applications. This section will delve into two major prospects in this field: the potential in gene editing and expanding therapeutic applications.
Potential in Gene Editing
mRNA technology offers intriguing possibilities in gene editing. Utilizing mRNA as a vehicle for delivering CRISPR/Cas9 components allows for a more precise approach to genetic modification. Traditional methods, which often use plasmids or viral vectors, can sometimes lead to off-target effects. In contrast, mRNA can be engineered for transient expression, leading to reduced risks of unwanted mutations.
This approach enables a more controlled activation of the CRISPR mechanism. Potential applications include:
- Targeted Therapies: In treating genetic disorders, targeted therapies can correct mutations at specific loci.
- Research Applications: mRNA delivery can facilitate real-time studies of gene function and regulation, enhancing our understanding of complex biological systems.
- Ethical Considerations: Utilizing mRNA may also address ethical concerns related to stability and potential long-term effects associated with other gene editing techniques.
Expanding Therapeutic Applications
The scope of therapeutic applications using mRNA technology is vast. Historically, mRNA has gained attention primarily for its role in vaccine development, especially highlighted during the COVID-19 pandemic with vaccines like Pfizer-BioNTech and Moderna. However, the future holds promise for expanding mRNA applications beyond vaccines. This includes:
- Cancer Treatment: mRNA can help produce tumor-associated antigens, promoting the immune system's recognition and destruction of cancer cells. This could lead to the development of personalized cancer immunotherapies.
- Protein Replacement: In disorders where a specific protein is lacking, mRNA can be employed to instruct cells to produce the deficient protein.
- Genetic Disorders: Scientists are exploring ways to provide cells with the necessary instructions to produce missing or dysfunctional proteins. This could revolutionize approaches for conditions like cystic fibrosis or muscular dystrophy.
"The adaptability of mRNA technology opens doors to previously unapproachable therapeutic realms, highlighting its transformative potential in modern medicine."
As researchers continue to study mRNA dynamics, it's apparent that the future directions in this field may well redefine our approaches to disease treatment, prevention, and understanding of the human genome. The realization of these potential goals hinges upon interdisciplinary collaboration and innovative thinking, making it a robust area of ongoing research.
Finale
The conclusion is a fundamental part of this article, drawing together all discussed elements around messenger RNA (mRNA). mRNA serves as a vital component in cellular biology, paving the way for advancements in genetics and medicine. Summarizing the key points are necessary to encapsulate the importance of mRNA in a clear and concise manner. It supports understanding of both basic concepts and complex applications, particularly in therapies and vaccines.
Summary of Key Points
- mRNA is crucial for protein synthesis, connecting genetic information and functional expression.
- Its role in transcription and translation outlines the dynamic processes of gene expression and regulation.
- The contrast between mRNA and DNA elucidates their functions, with mRNA being the active messenger in protein production.
- Biotechnology harnesses mRNA capabilities, including innovative therapeutic agents and vaccine developments, notably seen in the response to COVID-19.
- Research advances point toward expanding the applications of mRNA beyond current uses, such as in gene editing.
- Challenges in stability and ethical concerns highlight the complexities surrounding mRNA therapeutics.
Final Thoughts on mRNA's Impact
The impact of mRNA extends beyond the laboratory, influencing clinical practices and public health policies. Its significance has become more evident through recent global health challenges, showcasing its potential swiftly. The implications in personalized medicine and gene therapies exhibit promise, opening doors for future health innovations. As we continue to explore mRNA's potential, ongoing research and technological advancements will be critical. These innovations will likely shape how we approach medical treatments and disease prevention moving forward.
"Understanding mRNA provides insights that are crucial for the future of medicine, driving change in how we treat illnesses and respond to health challenges."
mRNA holds the key to transforming our biological understanding and therapeutic capabilities. It is clear that the research on mRNA will remain an area of focus, providing numerous opportunities and challenges in the quest to improve human health.