Exploring the Working Volume of 24-Well Plates
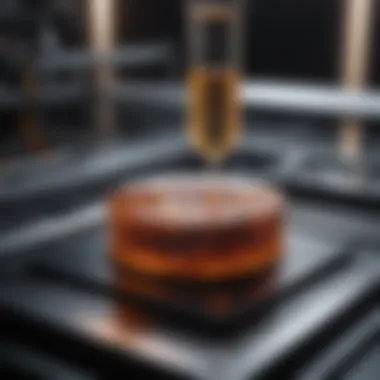
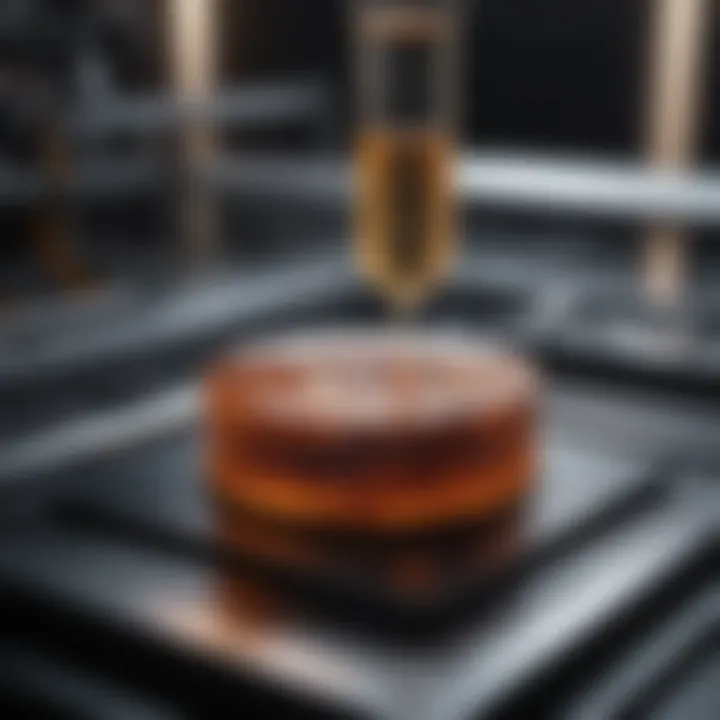
Intro
In the realms of laboratory research, the tools we use can often dictate the outcomes of our experiments, and 24-well plates are no exception. These small, flat, plastic trays with multiple wells have become mainstays in biological and chemical research settings. As their name suggests, each plate contains 24 individual wells that researchers utilize for various applications, from cell culture to molecular assays.
Understanding the working volume of these plates becomes crucial, as it directly influences the efficiency and accuracy of experiments. The working volume refers to the volume of liquid that researchers can effectively utilize in each well without risking evaporation or overflow, maximizing assay performance. The careful consideration of this volume is paramount in ensuring precise experimental results.
In this article, we aim to dive deep into the nuances of working volume associated with 24-well plates, unpacking the dimensional specifications and calculations that underpin them. We'll also discuss how understanding this aspect can enhance research methodologies and offer comparative insights with other multi-well formats.
Key Concepts and Terminology
Definition of Key Terms
Before jumping into the details, it’s essential to clarify a few key terms that will pop up throughout the discussion:
- Working Volume: The maximum volume of liquid that can be accommodated in each well without causing spillover or excessive evaporation.
- Total Volume: The entire volume capacity of a well, typically slightly larger than the working volume to account for headspace and evaporation.
- Evaporation Rate: The rate at which liquid evaporates from the well, influenced by factors like temperature, surface area, and humidity.
Concepts Explored in the Article
In our exploration, we’ll address the following concepts:
- Dimensions and Specifications: Discussing the size and design of 24-well plates and how these elements affect their working volume.
- Calculation of Working Volume: Providing formulas and methodologies for accurately determining the working volume across different experimental setups.
- Applications: Examining the importance of working volume in various scenarios, including drug testing, enzyme assays, and other biological experiments.
- Comparison with Other Formats: Offering insights into how 24-well plates compare with other multi-well formats, like 96-well and 384-well plates.
"The success of your experiment often starts with an understanding of your tools."
Findings and Discussion
Main Findings
Through this article, it becomes clear that the working volume of 24-well plates is not merely a number; it holds significance in experimental design. For one, many laws of fluid dynamics come into play. For instance, researchers should consider that a higher working volume might lead to increased evaporation rates, potentially skewing results. Conversely, undershooting the volume necessary for a reaction could impede outcomes as well.
Potential Areas for Future Research
While current literature provides a foundation, there are still areas ripe for exploration. Future research could delve into:
- Impact of Different Materials on Working Volume: Exploring how variations in plate materials affect evaporation rates.
- Optimization Techniques: Investigating strategies to minimize evaporation and enhance assay robustness.
- Longitudinal Studies: Analyzing how changing laboratory environmental conditions affect working volume over extended periods.
Prelims to 24-Well Plates
In the realm of laboratory research, 24-well plates stand as a vital tool for various experimental procedures. Understanding their design and functionality is key for any researcher who wishes to maximize the potential of their experiments. These plates, typically made from high-quality plastics, provide a standardised format that facilitates the growth of cells, screening of compounds, and an array of assays that explore biological interactions.
The significance of 24-well plates goes beyond their sheer utility; they play a pivotal role in experimental consistency. When planning any experiment, the ability to control variables such as volume, cell density, and incubation conditions becomes paramount. Researchers find that 24-well plates not only simplify these processes but also enhance their ability to obtain reproducible results. By honing in on the nitty-gritty of well dimensions and working volume, scientists can better tailor their methodologies to suit specific experimental requirements, thus improving both efficiency and precision.
Definition and Purpose
A 24-well plate, as the name suggests, features 24 individual wells arranged in a 6 x 4 format. Each well typically has a volume ranging from 1 to 3 milliliters, depending on the manufacturer and design specifications. The primary purpose of these plates is to provide a controlled environment where cells or other biological samples can be maintained. Moreover, their compact size makes them economical, allowing multiple experiments to run concurrently without taking up too much lab space.
Ultimately, the 24-well plate serves as an effective platform for both qualitative and quantitative analysis in various fields of study, including microbiology, pharmacology, and molecular biology. By delineating the spatial organization within each well, these plates support targeted experiments involving different treatments or conditions, thereby driving the research process forward.
Applications in Laboratories
The versatility of 24-well plates can be seen across a wide swath of laboratory applications. Here are a few significant uses that highlight their flexibility:
- Cell Culture: One of the foremost applications is the cultivation of various cell types. Researchers use these plates for amassing cell lines in controlled conditions, enabling experiments in toxicology and pharmacodynamics.
- Compound Screening: In drug discovery, the plates are instrumental for high-throughput screening. They allow multiple compounds to be assessed simultaneously against biological targets, accelerating the pace of research significantly.
- Enzyme Assays: With the right substrate and conditions, 24-well plates can be utilized for enzyme activity assays, making it easier to compare results across compounds and concentrations.
- PCR: Lastly, in molecular biology, these plates can support Polymerase Chain Reaction setups. They do this while allowing for quick thermal cycling, necessary for amplifying DNA.
Physical Characteristics of 24-Well Plates
Understanding the physical characteristics of 24-well plates is critical for researchers aiming to achieve precision in their experiments. These characteristics dictate how the plates will interact with various substances, affecting factors like sample volume, reaction rates, and overall experiment reproducibility. They serve as the backbone of any experimental design that employs these versatile tools. When you grasp these fundamental aspects, you place yourself in a better position to optimize your methodologies and results.
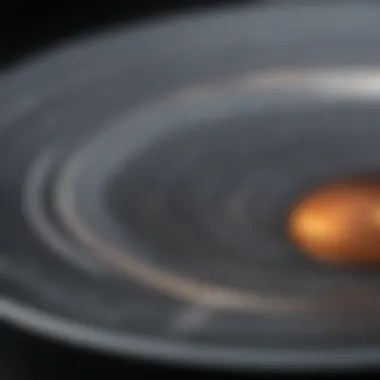
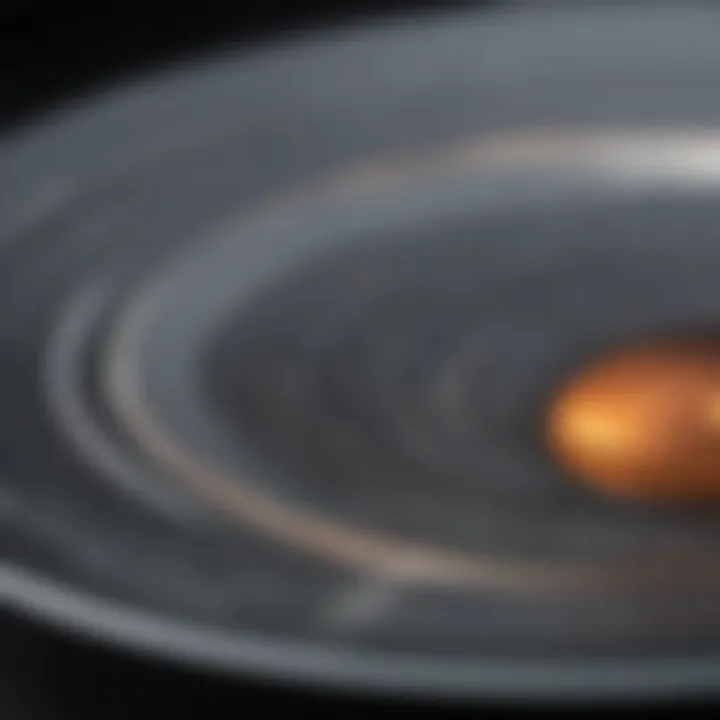
Material Composition
The material from which a 24-well plate is made can greatly influence its application and performance in the lab. Most commonly, these plates are constructed from polystyrene or polycarbonate polymers, each possessing unique properties.
- Polystyrene:
This is the most widely used material, as it is crystal clear, allowing easy visual access to the samples. Polystyrene plates are typically ideal for cell culture, providing appropriate surface characteristics for cell adhesion. They are also inexpensive and readily available. - Polycarbonate:
While more durable than polystyrene, polycarbonate plates are often utilized in applications requiring high temperature or chemical resistance. They may not be as optically clear as polystyrene but offer enhanced durability for rigorous testing environments.
"The choice of material goes hand-in-hand with the intended experimental outcomes."
Understanding the nuances of these materials aids in the selection process for your specific needs. The reactivity of the materials with your samples and solvents can impact the integrity of your results.
Dimensions and Volume Specifications
The dimensions and specifications of 24-well plates often dictate their compatibility with specific laboratory protocols. Each well typically has a standard diameter of about 16 mm and a total volume ranging from 1.5 to 3.0 ml, but this can vary based on the specific type of plate being used.
- Dimensions:
The standard layout of a 24-well plate allows for efficient use of space and easy management of numerous samples simultaneously. This makes it possible to carry out comparative analyses with minimal effort. - Volume Capacity:
Understanding the volume capacity of each well is essential when planning experiments. The so-called "working volume"—the amount of liquid the well can hold effectively—generally should not exceed the total capacity. This enables thorough mixing of samples without risk of overflow or contamination.
Moreover, keen attention should be given to how much of the total volume is practical to use. For instance, using too little could lead to inaccuracies in experimental results, while excessive volumes may result in waste and complicate the process without improving outcomes.
In this way, dimensions and volume specifications are not just numbers and measurements; they have direct implications on lab operational efficiency and experimental accuracy. Understanding these characteristics enhances the overall utility of 24-well plates in scientific research.
Understanding Working Volume
The working volume of a 24-well plate is an essential factor that shapes the approach researchers take in experimentation. Having a clear definition and grasping its significance can greatly aid in the optimization of experimental designs and outcomes. Working volume refers to the amount of liquid that can be effectively utilized in each well, creating a vital connection between the method of experimentation and the reliability of results.
Definition of Working Volume
Working volume is often simply defined as the usable liquid volume within the wells of a plate, taking into account factors such as the height of the liquid column and the well's dimensions. In a standard 24-well plate, this volume typically varies, commonly falling between 0.5 ml to 3 ml per well, depending on the experimental needs and design.
However, merely having a numerical value isn't sufficient. Researchers must consider the liquid dynamics involved, where meniscus formation and evaporation can factor subtly into the liquid's effective volume. Thus, defining working volume must also account for how these elements interact in a practical lab setting.
Importance in Experimental Design
Understanding the working volume isn't just an academic exercise; it has practical implications that can make or break an experiment. Here are a few key reasons why:
- Precision and Accuracy: Accurate volume measurements lead to more reliable results. When volumetric errors occur, they can impact concentrations of reactants, affecting outcome validity.
- Surface Area to Volume Ratio: Different volumes can influence the interaction of substances. For instance, smaller working volumes can enhance diffusion rates due to increased surface area exposure, while larger volumes may dilute reactions, impacting kinetic studies.
- Resource Management: Conscious selection of working volume can optimize reagent use, minimizing waste and reducing costs, especially in high-throughput scenarios.
- Scalability of Results: Larger working volumes may help extrapolate findings to larger-scale applications while maintaining consistent conditions across wells.
"Selecting the right working volume is like picking the right tool for a job - it directly impacts effectiveness and efficiency."
To make the most of experimental designs, researchers can refer to guidelines and previous studies that explore various scenarios of working volume. This is critical in fields such as biochemistry or molecular biology, where precise conditions often dictate the success of a procedure.
Overall, understanding working volume is foundational in laboratory settings. With this knowledge, students, researchers, and professionals can navigate the complexities of experiments, ensuring higher reliability and reproducibility in their scientific endeavors.
Calculating the Working Volume
Calculating the working volume of a 24-well plate is an essential step for anyone engaged in lab work. This number directly influences how experiments are executed and determines the effectiveness of the results obtained. If researchers neglect precise volume calculations, it could result in skewed data or unrepeatable experiments. Thus, mastering this calculation enables scientists to refine their experimental designs, making reliable comparisons between different trials.
Standard Measurements
In essence, the working volume refers to the effective liquid volume held in each well of the plate that contributes to experimental work. Typically, a standard 24-well plate has a maximum total volume ranging from 1 to 3 milliliters per well, depending on the manufacturer specifications and shape. However, the actual working volume can differ significantly. Generally, researchers aim for a working volume that is less than the maximum capacity to prevent spillovers that can taint neighboring wells.
The calculation of working volume goes as follows:
- Identifying Maximum Volume: Understand what the limit is for your specific well plate.
- Determining Working Volume: Establish an appropriate volume that takes into account the experimental setup. Common guidelines suggest filling to 300-800 microliters, but this varies based on intended use.
It must be noted that handling methodologies, like aspiration and dispersion, are also tied to these measurements.
Factors Influencing Volume Calculation
Calculating the working volume isn't just about knowing the numbers; several factors come into play which can significantly affect these calculations. Here are some worth considering:
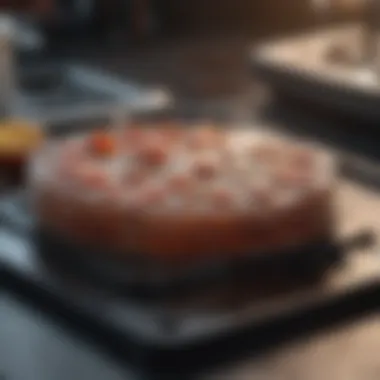
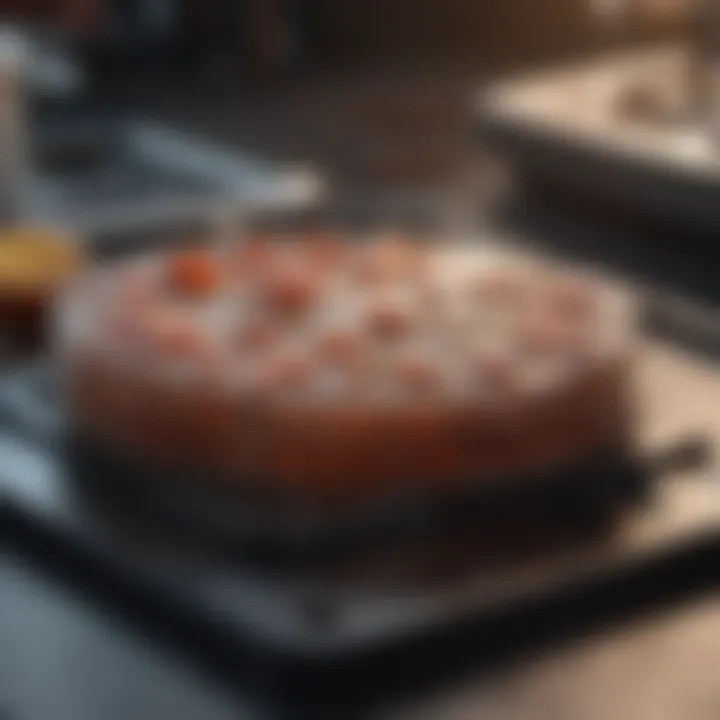
- Evaporation Rates: In longer experiments, solvent loss through evaporation will directly affect volumes and concentrations.
- Surface Tension: The behavior of liquids in the wells, influenced by surface tension, can alter how much of the solution remains effective for reactions.
- Reagents Used: Different solutions have varying densities and properties. An aqueous solution versus an oil-based reagent may have completely different volumetric behavior.
- Temperature Fluctuations: The temperature can affect the volume due to expansion or contraction, impacting the precise measurement one aims for.
"Getting the calculations right is half the battle at the bench. Small miscalculations can lead to effects cascading down the line, resulting in flawed conclusions."
Comparison with Other Multi-Well Formats
The choice of a 24-well plate can significantly impact experimental design, especially when considering comparisons with other multi-well formats. Understanding how these plates stack up against their counterparts isn't just academic; it’s a practical necessity for many researchers. The variations in plate types allow for flexibility in experimental setups, tailored to specific conditions and requirements. Each format possesses characteristics that can either benefit or limit the outcomes of experiments.
Differences in Working Volumes
When examining different multi-well formats—often found in laboratories—what stands out are their working volumes. A 24-well plate typically allows for a working volume ranging anywhere from 0.5 to 3 milliliters, depending mainly on the intended application. In contrast, formats like 96-well plates have a smaller well capacity, usually capped at about 0.2 to 0.5 milliliters, which can alter the scalability of procedures.
Furthermore, larger plates such as the 6-well or 12-well plates facilitate greater working volumes, at times exceeding 5 milliliters. This great variation means that when planning experiments, researchers must be mindful of how the working volume could influence the dilution factors or concentration of reactants involved.
To illustrate:
- 24-well plates: 0.5 to 3 mL working volume
- 96-well plates: 0.2 to 0.5 mL working volume
- 12-well plates: Approximately up to 5 mL working volume
- 6-well plates: Generally can hold 10 mL or more in each well
This disparity is crucial for experiments that require precise control over conditions, as smaller volumes might lead to quicker evaporation rates and altered concentrations. Knowledge of these differences allows researchers to strategize effectively regarding their experimental needs.
Suitability for Various Applications
Choosing the right multi-well format hinges on the specific experimental needs at hand. The 24-well plate shines in a variety of applications, striking a balance between capacity and space efficiency. It's particularly favored in biological assays, such as cell culture and functional tests, where mid-range working volumes are advantageous for dilution or sample preparation.
For instance, if a team is working on a drug screening project, a 24-well plate allows for the testing of multiple compounds while affording enough volume to monitor responses over time. Moreover, for gene expression studies or protein assays, this format provides sufficient area to apply varied treatments across replicates.
In contrast, smaller formats like 96-well plates may be ideal for high-throughput screenings, while large formats, such as 6-well plates, might be better suited for experiments requiring more extensive interactions, such as maintaining larger cell mass or more significant quantities of reagents.
"The ability to efficiently choose between multi-well formats not only enhances experimental design but also impacts the quality of scientific inquiry."
Choosing the right plate not only maintains the integrity of individual experiments but also harmonizes with broader goals of research productivity and reliability. Thus, understanding your specific application can lead to a more strategic selection of a multi-well format, reducing waste and maximizing efficiency.
Impacts of Working Volume on Experiments
The working volume of a 24-well plate is more than just a number; it’s crucial in shaping the reliability and accuracy of experimental results. When researchers talk about working volume, they’re referring to the liquid that is actually utilized in any given well for their assays or experiments. Understanding how this volume impacts biological, chemical, or analytical responses can radically change the way one approaches experimental design.
Effects on Reactivity and Results
When it comes down to it, the working volume influences the reactivity of the components involved in a reaction. In a smaller volume, the concentration of reactants tends to be much higher compared to larger volumes. This can cause reactions to proceed at a different rate, which could be perfectly fine or might lead to unexpected results. For instance, consider enzymatic assays where the enzyme efficiency is often linked to substrate concentration. In the case of a diminished working volume, the substrate could become saturated, affecting the reaction kinetics.
Moreover, when solutions are diluted too much due to an inappropriate working volume, critical interactions might not even take place, leading to lower rates of reaction or reduced sensitivity in assays. The chemical environment in which reactions occur becomes essential in predictive modeling for results. If too much volume is used, compounds that are troublesome in a reaction could overwhelm desired interactions or even interfere with analytic signals.
In summary, the size of the working volume can tip the scales dramatically in favor of one outcome over another.
Influence on Reproducibility
Reproducibility is the heartbeat of scientific research. If experiments cannot be replicated, their credibility suffers. Working volume plays a significant role here as well. Each time an experiment is conducted, consistent volumes can lead to uniform results. However, say you’re using a 24-well plate with varying amounts of liquid across the wells – the results will likely be inconsistent, muddling what could have been an otherwise straightforward experiment.
Small variations in working volume can lead to larger variations in the final measurement. Think of pipetting errors. If one well has a slightly higher volume due to miscalculation, not only does that change the concentration, but it can also significantly affect biochemical processes happening within that well, ultimately skewing data.
To make it more concrete, in a scenario where pharmaceuticals are being screened, even minor discrepancies in volumes can lead to significant differences in observed pharmacodynamics or potency.
In essence, to achieve reliable and reproducible results that can withstand scrutiny, maintaining strict control over working volume during experiments is non-negotiable. Engaging with this aspect—considering how changes in working volume can affect results and reproducibility—creates a more robust framework for scientific inquiry.
"Precision in working volume leads to clarity in experimental outcomes."
By aligning the working volume with the specifics of your experiment, researchers can enhance predictability and reliability in outcomes. Understanding these nuances is key in moderner experimental designs and significantly impacts the validity of research findings.
Optimizing Working Volume
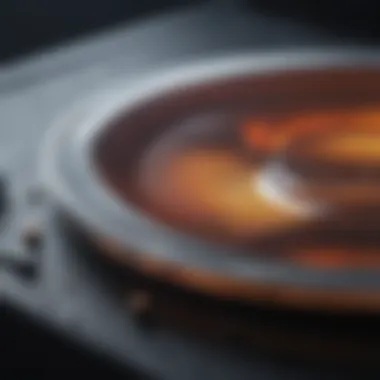
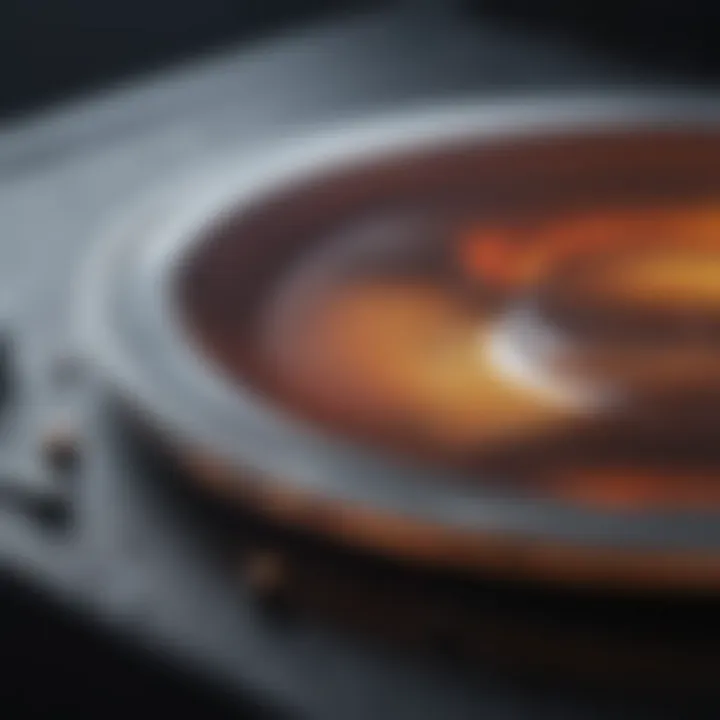
Optimizing the working volume in a 24-well plate is like fine-tuning a musical instrument; it’s all about finding the right balance to achieve the best results. Research settings often demand strict accuracy, and how researchers manage the volumes in each well can greatly affect the entire experiment. This section highlights the intricacies involved in optimizing working volume, laying out specific elements to consider, the benefits derived from careful adjustments, and the implications of such modifications on experimental outcomes.
Adjusting Volumes for Specific Experiments
When delving into the realm of 24-well plates, adjusting volumes for specific experiments becomes paramount to ensure effective results. Each experiment is unique, and the requirements for each can differ vastly — be it cell culture, drug screening, or assays. Each application can demand different liquid volumes, driven by several factors:
- Cell Type: Different cells have varied nutrient and growth factor requirements. For example, a well filled with a high number of adherent cells may need less volume since the cells will proliferate and cover the well surface faster.
- Experimental Conditions: Temperature, chemical sensitivity, and other environmental parameters can change how much liquid is optimal. For instance, a highly volatile solution might need tighter controls in volume to minimize evaporation over time.
- Detection Methods: Certain assays, like fluorescence or luminescence, may require precise volumes for optimal signal generation. Too much or too little liquid can wash out the signal, leading to misleading results.
By understanding these elements, researchers can tailor their liquid volumes per experiment, aligning with what works best for their specific protocols. Adjustments might require trial and error, but they ultimately lead to a more refined and reliable data set.
Balancing Resource Use and Results
In the fast-paced world of scientific research, balancing resource usage with obtaining valid results is a constant juggle. Working with a 24-well plate can sometimes lead to a more resource-intensive approach if not handled optimally. Balancing this involves a few key considerations:
- Cost-Effectiveness: Materials in lab experiments can add up quickly. By optimizing the working volume, researchers can cut costs associated with reagents or samples. For instance, using precisely the right amount of a growth factor minimizes waste.
- Time Efficiency: More often than not, working with less liquid allows for quicker handling and easier manipulation of samples. This shift can speed up the experimentation timeline significantly.
- Consistent Data Yield: Maintaining a balance ensures consistent data collection. If volumes are adjusted haphazardly, inconsistencies may arise, throwing into question the reproducibility of results. Measurements should always reflect the volumes believed to yield the best outcomes.
By keeping an eye on both resource use and achievability of results, scientists can not only maintain integrity in their work but also foster a more sustainable approach to research practices.
Overall, optimizing working volume in a 24-well plate isn’t just a technical consideration; it’s part of an overarching strategy that impacts the efficiency, cost, and validity of research experiments. Getting the volumes right means more than just adhering to protocols; it’s about enhancing scientific inquiry and pushing the boundaries of knowledge effectively.
Future Directions in plate Design
As we forge ahead in the realm of laboratory technologies, the evolution of plate design stands out as a remarkable area of innovation. The working volume in 24-well plates is a hot topic, driving researchers and manufacturers alike to consider how adjustments can enhance experimental efficiency and accuracy. Understanding future directions in plate design is vital, as it shapes not just the methodology but also the quality of research outcomes. Contemporary demands in biological applications necessitate a radical rethink of traditional designs, and innovations are emerging as the keystone in this transformation.
Innovations in Plate Technology
Several notable innovations are surfacing in plate technology that could revolutionize the way laboratories conduct experiments. For instance, multi-layered plates are gaining traction; these incorporate different materials, affecting properties such as permeability or chemical stability. Such advancement might allow parallel optimization of various conditions within the same experiment, saving time and resources.
Moreover, some manufacturers are exploring smart plate technology, which includes embedded sensors that monitor environmental factors like temperature and pH in real time. This modernization offers insights that traditional methods simply cannot. Imagine adjusting experimental parameters based on immediate feedback rather than waiting for results post-experiment; this could tremendously elevate the speed and reliability of data collection.
Furthermore, 3D printing technologies are also making waves, enabling bespoke plate designs tailored to specific experimental needs. This could benefit niche research areas where standard plates fall short. Customization in design allows for better integration of experimental variables, paving the way for enhanced accuracy in results.
- Multi-layered plates for varied conditions.
- Smart technology for real-time monitoring.
- 3D printing for custom designs.
Potential Impact on Research
The implications of these advancements in plate design can’t be overstated. As innovations progress, researchers can anticipate richer data collectio and finer control over experimental factors. Plates designed for specific applications could increase reproducibility across different labs, eliminating discrepancies caused by traditional materials that may react variably under certain conditions.
Additionally, the flexibility of design may also push the diision of tasks within research teams. This ability to tailor plates for diverse applications means that collaboration among varied disciplines could become more streamlined. Biologists could work more closely with engineers to develop the precise tools needed for advancing research in areas like drug discovery or genetic studies.
"The future of laboratory experiments lies not just in what we investigate but also in how we design the tools that help us in that quest."
Moreover, by utilizing these advanced plate technologies, researchers will likely achieve higher throughput in experimental run times, ultimately contributing to faster iterations of testing and exploration. As scientific inquiry pushes the boundaries of knowledge, the need for agility and adaptability in lab equipment continues to grow.
Culmination and Implications
In the realm of laboratory research, understanding the intricacies of working volume in a 24-well plate elevates both experimental design and outcome evaluation. The insights gathered from this exploration serve not only to inform current practices but to pave the way for improved methodologies in future research. The relationship between working volume and experimental integrity cannot be overstated; it directly influences the conditions under which reactions or assays occur, thereby determining the reliability of the results obtained.
Among the specific elements that emerge from this study, the precision in calculating working volume stands out. Accurate calculations lead to more reliable data, which is the cornerstone of research credibility. Furthermore, comprehending the impacts of volume adjustments on biological assays and chemical reactions allows researchers to fine-tune their experiments for maximum efficacy. This is not merely a technical consideration but a fundamental aspect that can make a meaningful difference in outcomes, especially in fields where data integrity is paramount.
The implications extend beyond just the practical aspects of conducting experiments. As laboratories continuously strive for innovation and reproducibility, understanding working volume can facilitate the development of best practices and protocols that align with the evolving demands of research. Efficient use of resources, coupled with a firm grasp of volume considerations, can significantly enhance experimental designs and, ultimately, the scientific inquiry process.
"In science, what we want are some experiments which will give us just one result, but a clear one. Working volume can help achieve this clarity."
Summary of Key Points
- The working volume in 24-well plates directly affects experimental outcomes.
- Accurate calculations are essential for data reliability and reproducibility.
- Understanding volume adjustments enables tailored experimental designs.
- Implications for best practices and resource management are significant.
Final Thoughts on Working Volume Usage
As we concluded this examination, it becomes clear that the significance of working volume transcends mere numbers in a lab notebook. Each drop or microliter carries the weight of potential discoveries and advancements in scientific understanding. Researchers should approach working volumes not as an afterthought but as a critical component of experimental planning.
Moving forward, engaging with new innovations in plate design may unlock avenues for optimizing working volume further, allowing for more streamlined workflows and enhanced data quality. Keeping abreast of advancements in this area can sharpen a researcher's competitive edge, enabling them to navigate the ever-complex landscape of scientific inquiry intelligently.
In summary, a deep understanding of the working volume helps researchers not just succeed in individual experiments but also contribute to a broader mission of enhancing the credibility and reliability of scientific research.