The Synthesis and Applications of Carbon Nanotubes
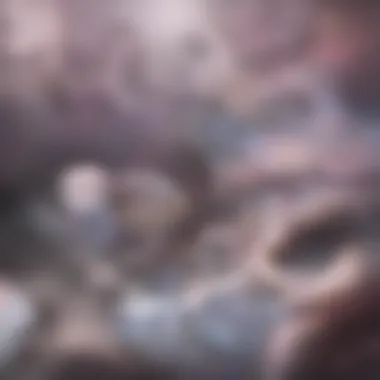
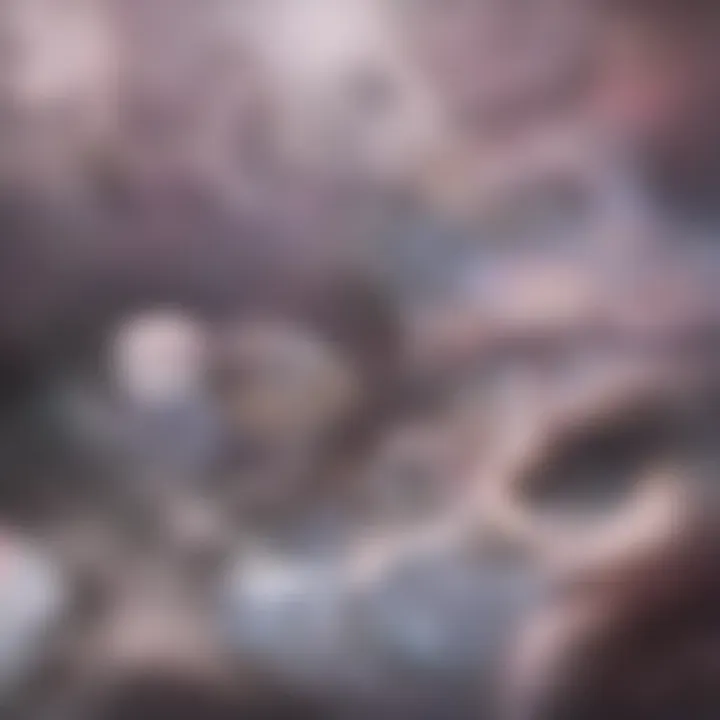
Intro
Carbon nanotubes (CNTs) have emerged as a revolutionary material in various fields of science and engineering. Their unique structure, characterized by cylindrical arrangements of carbon atoms, endows them with remarkable electrical, thermal, and mechanical properties. The significance of CNTs is made evident through their diverse applications, ranging from nanotechnology to advanced materials science. This article delves into the synthesis methods of carbon nanotubes and explores the implications of their unique characteristics.
Understanding the synthesis of CNTs requires a grasp of certain key concepts and terminology. The processes involved in producing these nanostructures not only determine their properties but also affect their performance in practical applications.
Key Concepts and Terminology
Definition of Key Terms
- Carbon Nanotubes (CNTs): Cylindrical nanostructures comprising carbon atoms arranged in hexagonal lattices. They can exhibit either single-walled (SWCNTs) or multi-walled (MWCNTs) configurations.
- Chemical Vapor Deposition (CVD): A widely used technique for synthesizing CNTs. It involves the chemical reaction of gaseous precursors on a substrate to form solid material.
- Laser Ablation: A method that utilizes high-powered laser beams to vaporize carbon-containing materials, allowing CNTs to form as the vapor cools.
- Arc Discharge: A technique where an electrical discharge is created between two carbon electrodes, leading to the formation of CNTs through the resulting plasma.
Concepts Explored in the Article
The article explores various synthesis techniques in detail, examining how each method contributes to the characteristics and applications of the resulting carbon nanotubes. Readers will gain insights into the benefits and drawbacks of each approach and their implications on the practical use of CNTs.
Findings and Discussion
Main Findings
The synthesis methods play a crucial role in determining the quality and functionality of carbon nanotubes. Key findings include:
- CVD is favored for producing high-quality CNTs on a large scale, given its controllable parameters and efficiency.
- Laser ablation offers a means of creating high-purity CNTs, although it is less scalable due to equipment limitations.
- Arc discharge, while effective, often results in a mixture of CNTs and other carbon products, making purification necessary.
Potential Areas for Future Research
Future investigations can explore innovative methods to enhance synthesis processes and the scalability of CNT production. Consideration should also be given to:
- Evaluating the environmental impact of CNT synthesis.
- Investigating the possibilities for functionalizing CNTs to tailor their properties for specific applications.
- Studying the long-term stability and reproducibility of CNT performance in real-world applications.
"The versatility of carbon nanotubes underscores their significance in the advancement of modern materials science and nanotechnology."
By synthesizing the information drawn from various synthesis techniques, this article aims to provide a comprehensive understanding of carbon nanotubes. As students, researchers, educators, and professionals read through the article, it will illuminate the relevance and applications of these remarkable nanostructures.
Prelude to Carbon Nanotubes
The exploration of carbon nanotubes is crucial because these materials exhibit unique characteristics that bridge the fields of nanotechnology, materials science, and electronics. Understanding carbon nanotubes begins with recognizing their remarkable properties and potential applications. This article intends to delve into the synthesis and utility of carbon nanotubes, illuminating their role in contemporary science.
Definition and Structure
Carbon nanotubes (CNTs) are cylindrical nanostructures composed purely of carbon atoms, arranged in a hexagonal lattice similar to graphite. Each cylinder can have one layer (single-walled nanotubes) or multiple layers (multi-walled nanotubes).
Their structure is essential, as the arrangement of carbon atoms determines their specific properties. For instance, single-walled nanotubes often demonstrate exceptional electrical conductivity due to their unique band structure, which varies based on the arrangement of carbon atoms.
The diameter of these tubes can be just a few nanometers, while their length can reach micrometers or longer. This small scale provides them a high surface area-to-volume ratio, enhancing their strength and conductivity. Notably, the chirality of the nanotube, which refers to the twist in the arrangement of carbon atoms, defines whether a nanotube behaves as a metal or semiconductor.
Historical Context
The concept of carbon nanotubes emerged in 1991, following their discovery by Sumio Iijima. However, the groundwork for understanding these structures was laid through advancements in fullerene research in the 1980s. Early experiments focused mainly on the synthesis of fullerenes, a form of carbon molecule, leading eventually to the isolation and analysis of carbon nanotubes.
Research in the 1990s marked a significant advancement in comprehending nanotube properties. Investigations into their mechanical strength, thermal conductivity, and electrical properties began to illustrate potential applications. By the early 2000s, studies opened pathways for applications in various sectors, including electronics and materials science, leading to innovative uses in future technologies.
As the relevance of carbon nanotubes expanded, researchers faced challenges such as scalability in production and purity control. Yet, the persistence of scientific inquiry into carbon nanotubes has made them a focal point in nanotechnology, transforming industries and academic research alike.
Carbon nanotubes stand at the intersection of materials science and nanotechnology, offering transformative potential across multiple domains.
Properties of Carbon Nanotubes
Understanding the properties of carbon nanotubes (CNTs) is crucial because these characteristics directly contribute to their functionality in various applications. The remarkable qualities of CNTs have captured the attention of researchers and professionals alike. Basically, these properties can be grouped into three main categories: electrical conductivity, mechanical strength, and thermal conductivity. Each of these traits plays a significant role in the potential applications of CNTs across different fields, including electronics, materials science, and medicine.
Electrical Conductivity
Carbon nanotubes exhibit unique electrical properties that depend largely on their structural configuration. Depending on how the atoms are arranged, CNTs can exhibit either metallic or semiconducting behavior. This versatility stems from their quantum properties, allowing them to conduct electricity with minimal resistance. The high electrical conductivity of CNTs makes them attractive for applications in nanoelectronics. They can be used to develop faster, smaller, and more efficient transistors, potentially leading to a new era of electronic devices that outperform traditional silicon-based counterparts. Researchers continue to explore their use in conductive polymers and other materials to enhance electrical performance, pushing the boundaries of current technology.
Mechanical Strength
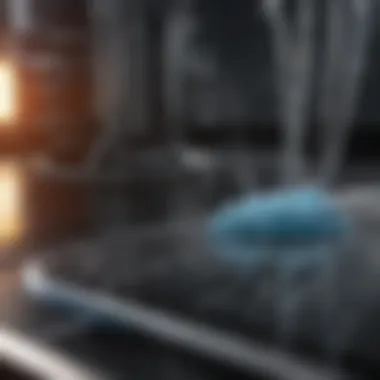
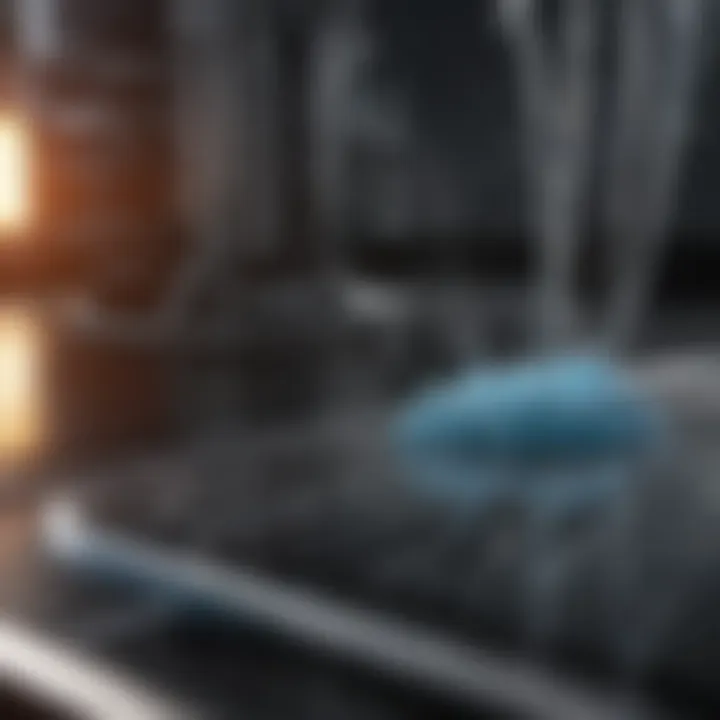
One of the most impressive aspects of CNTs is their mechanical strength. These materials display a tensile strength higher than that of steel while remaining significantly lighter. This property is attributed to their unique cylindrical structure and carbon-carbon bonding. In practice, CNTs can strengthen composite materials, offering improved durability and resilience without adding substantial weight. Applications in aerospace, automotive, and construction industries are particularly promising due to these mechanical properties. The challenge lies in integrating CNTs effectively into existing materials and ensuring uniform distribution to leverage their full strength.
Thermal Conductivity
Carbon nanotubes also showcase exceptional thermal conductivity, which is crucial for heat management in various applications. They can efficiently conduct heat along their length, making them ideal materials for thermal interface materials, heat sinks, and other applications requiring effective heat dissipation. This property supports their use in electronics, where overheating can be a significant concern. However, the thermal management capability of CNTs also invites exploration in fields like energy storage and conversion. As researchers invest time into understanding and enhancing the integration of CNTs in devices, the scope for innovation in thermal management solutions is boundless.
"The amalgamation of electrical, mechanical, and thermal properties makes carbon nanotubes a cornerstone of advanced material science and engineering."
Methods of Synthesis
The synthesis of carbon nanotubes is crucial in determining their characteristics and applications. Different methods yield various types of nanotubes with distinct properties. Understanding these methods provides insights into their scalability, cost, and effectiveness in producing high-quality carbon nanotubes. Each technique has its own advantages, challenges, and ideal use cases that will be covered in detail. The synthesis process essentially sets the stage for the later utilization of these nanotubes in diverse fields like electronics, materials science, and medicine.
Chemical Vapor Deposition
Chemical vapor deposition (CVD) is one of the most commonly used methods for synthesizing carbon nanotubes. This technique involves heating a substrate in the presence of carbon-containing gases, which decompose and precipitate carbon atoms on the substrate surface, forming nanotubes.
The benefits of CVD include:
- High yield: This method often results in a substantial amount of nanotubes within a short time frame.
- Control over morphology: By adjusting the gas composition and temperature, researchers can control the diameter and length of the nanotubes produced.
- Quality and purity: CVD often produces high-purity materials with fewer defects, which is essential for many applications.
However, there are also considerations such as the need for expensive equipment and the necessity of precise control over various parameters to achieve the desired results.
Laser Ablation
Laser ablation is another effective method for producing carbon nanotubes. In this technique, a laser is directed at a carbon target in an inert atmosphere. The intense heat generated vaporizes the target material, allowing carbon atoms to condense and form nanotubes.
Key aspects of laser ablation include:
- Production of high-quality nanotubes: This method typically yields high-quality carbon nanotubes due to the rapid cooling process.
- Flexibility in design: Researchers can modify the target material or conditions to create specific types of nanotubes, including single-walled and multi-walled structures.
- Controlled environment: The inert atmosphere prevents unwanted reactions and enhances the quality of the synthesized nanotubes.
On the downside, laser ablation can be more costly compared to other methods, limiting its scalability for large-scale production.
Arc Discharge
Another method to synthesize carbon nanotubes is arc discharge, where a carbon electrode generates an arc in an inert gas environment. This process causes carbon to vaporize and subsequently forms nanotubes during the cooling phase.
The advantages of arc discharge include:
- High-quality product: This method results in nanotubes with fewer defects, making them suitable for various applications.
- Single-walled nanotubes: Arc discharge is particularly known for producing high amounts of single-walled carbon nanotubes, which are highly sought after for their unique properties.
However, issues such as lower yield and the necessity for skilled handling make arc discharge less common in some settings than CVD or laser ablation methods.
Comparison of Methods
Comparing the methods helps clarify their respective strengths and weaknesses. Each synthesis method has implications regarding cost, complexity, and scalability.
- CVD is advantageous for high yield and control over properties but requires significant investment in equipment.
- Laser ablation allows for high-quality production but is costly for large-scale output.
- Arc discharge produces excellent quality and single-walled carbon nanotubes but faces challenges in yield and handling.
Understanding these methodsโ nuances can inform future research and commercial applications. Based on these considerations, choosing the suitable synthesis method aligns with intended applications, cost-efficiency, and desired properties.
Characterization Techniques
Characterization techniques are essential in the study of carbon nanotubes (CNTs). They enable scientists and researchers to understand the structural properties, purity, and functionality of these advanced materials. The effectiveness of CNTs in various applications hinges on their quality and structural integrity. There are several methods employed to characterize CNTs, each offering unique insights and benefits. Understanding these techniques helps in not only the evaluation but also the optimization of CNT production methods.
Scanning Electron Microscopy
Scanning Electron Microscopy (SEM) is a vital technique used for the surface analysis of carbon nanotubes. It provides high-resolution images that reveal the morphology and arrangement of CNTs on a substrate. SEM operates by scanning a focused beam of electrons across a specimen and detecting the resulting secondary or backscattered electrons. This interaction allows for the determination of the surface topography of the material.
Benefits of using SEM include:
- High resolution: Capable of providing images at sub-micrometer resolutions.
- Quick characterization: Rapid imaging offers significant time savings in research data collection.
- Versatile applications: Applicable to a wide range of samples, including both metals and non-metals.
However, there are considerations. The technique typically requires a conductive coating if the CNTs are non-conductive, which could potentially alter the specimen's properties. Nevertheless, SEM remains a preferred method for an initial overview of carbon nanotube structures and their distributions on surfaces.
Transmission Electron Microscopy
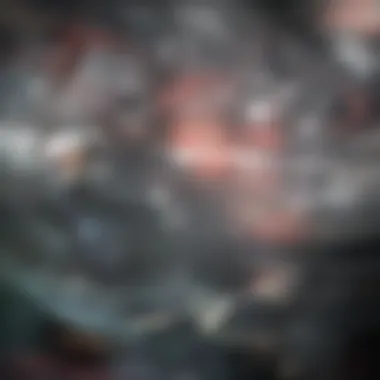
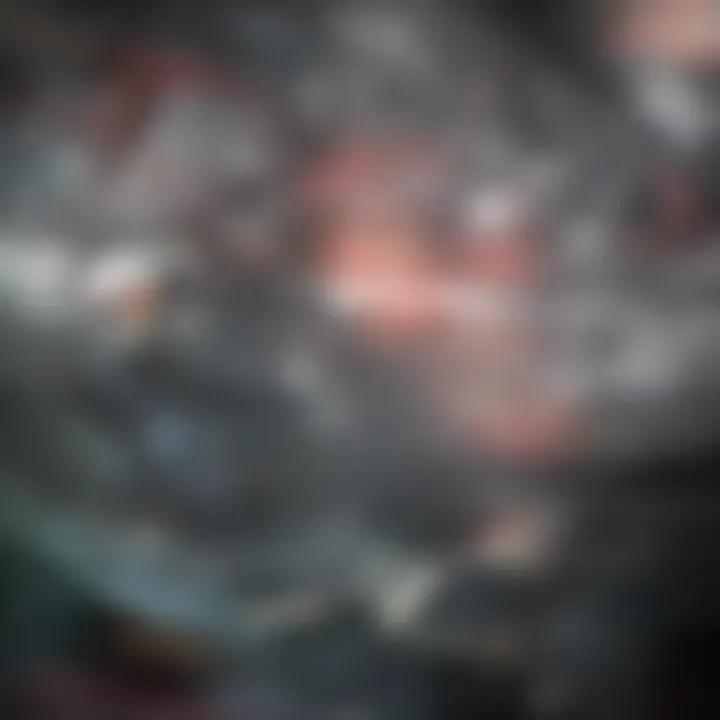
Transmission Electron Microscopy (TEM) provides intricate details about carbon nanotubes at atomic resolutions. Unlike SEM, TEM allows for the examination of internal structures. The principle of TEM involves transmitting electrons through a thin specimen. As the electrons pass through, they interact with the atoms in the material, generating an image based on electron scattering.
TEM is particularly useful for:
- Detailed analysis: It can visualize individual nanotubes and their cross-sectional structures.
- Defect characterization: Possible to identify defects or irregularities in CNTs that may impact their properties and applications.
- Crystallographic information: Provides insights into crystallinity and structural relationships.
Despite these advantages, TEM samples often require meticulous preparation that can be time-consuming. Furthermore, the vacuum environment necessary for TEM may affect delicate or unstable samples.
Raman Spectroscopy
Raman Spectroscopy is a non-destructive technique used to investigate the vibrational modes in carbon nanotubes. This method involves shining a laser on the sample and analyzing the scattered light. The frequency shift of the scattered light provides information about molecular vibrations, which can be directly correlated to the structure and quality of CNTs.
Key advantages include:
- Non-destructive analysis: Maintains the integrity of the CNT sample during examination.
- Sensitivity to defects: Can reveal subtle differences in structure, such as the presence of defects or functionalization.
- Quantitative assessment: Useful for determining the ratio of different carbon structures, such as the D band (defect band) to the G band (graphite band).
Applications of Carbon Nanotubes
The applications of carbon nanotubes (CNTs) are vast and significant, reflecting their unique properties and characteristics. CNTs exhibit remarkable strength, electrical conductivity, and thermal management capabilities. These attributes enable their use in various fields, fostering advancements in technology and improving existing materials. As researchers and industries continue to explore CNT applications, the benefits reveal a future where materials and designs become more efficient and sustainable.
Electronics and Optoelectronics
In the realm of electronics, carbon nanotubes are crucial components due to their excellent electrical properties. They exhibit high electrical conductivity and can function as semiconductors. This makes them suitable for various applications, including transistors and sensors. For instance, CNT field-effect transistors (FETs) have shown potential for high-speed and low-power applications. As the demand for faster and more efficient electronic devices grows, CNTs provide a pathway to meet these needs.
Their optoelectronic applications are also noteworthy. CNTs can absorb and emit light, making them suitable for light-emitting diodes (LEDs) and photovoltaics. The energy efficiency and performance of solar cells can be improved when CNTs are incorporated, enhancing the overall efficacy of energy generation technologies.
Material Science
In material science, carbon nanotubes have gained attention for their ability to reinforce materials. When added to composites, such as polymers and metals, CNTs can significantly enhance mechanical strength and reduce weight. This results in lighter, stronger materials that hold great potential in industries like aerospace and automotive.
Moreover, CNTs improve thermal conductivity, which can be beneficial in applications needing efficient heat management. As processes seek to optimize material use and energy consumption, CNTs represent a valuable asset that can lead to new designs and improved efficiency.
Medical Applications
The biomedical field has started to harness the potential of carbon nanotubes for various applications. One noteworthy area is drug delivery. Due to their nanoscale size, CNTs can transport medicines directly to targeted cells, improving the effectiveness of treatments while minimizing side effects. Their surface properties can be modified for enhanced biocompatibility, making them suitable for use in the human body.
Additionally, CNTs are explored for imaging and diagnostic purposes. They can be utilized as contrast agents in imaging techniques, leading to more accurate diagnosis and monitoring of diseases. Their multifunctional capabilities position them as valuable tools for advancing healthcare.
Environmental Applications
Environmental applications of carbon nanotubes hold promising potential as well. The unique structure and high surface area of CNTs enable them to adsorb various pollutants, making them effective in water purification and air filtration processes. This ability to remove contaminants could address significant environmental challenges and promote cleaner ecosystems.
In the area of energy storage, CNTs are being researched for use in batteries and supercapacitors. Their excellent conductivity and large surface area can enhance energy storage capacities, leading to longer-lasting and more efficient energy solutions. As sustainability becomes increasingly important, employing CNTs in energy solutions can play a critical role in shaping future technologies.
Challenges in Carbon Nanotube Production
The production of carbon nanotubes involves various challenges that can affect the properties and applications of these materials. Understanding these challenges is crucial for researchers and professionals in nanotechnology and materials science. Addressing issues surrounding purity, scalability, and health ensures that advancements in carbon nanotube applications can be achieved responsibly and effectively.
Purity and Quality Control
In synthesizing carbon nanotubes, maintaining purity is of paramount importance. Impurities can significantly alter the electrical, thermal, and mechanical properties of CNTs. High-purity nanotubes are essential for device applications, such as in semiconductors and composites. Common impurities include residual catalysts from growth processes, carbonaceous by-products, or even particles from the environment.
Quality control measures involve several techniques:
- Characterization Techniques: Employing methods like scanning electron microscopy and Raman spectroscopy allows for the analysis of CNT morphology and the detection of defects or impurities.
- Purification Processes: Using chemical treatment or flotation can help remove impurities post-synthesis. However, these processes must be carefully controlled to avoid damaging the carbon nanotubes themselves.
It is critical to establish standardized protocols for evaluating the purity of carbon nanotubes in order to ensure consistent performance across applications.
Scalability of Production Methods
Scalability presents another major challenge. Laboratory-scale synthesis techniques often do not translate well to industrial production. Current production methods like chemical vapor deposition, while effective, struggle with cost and efficiency when applied on a large scale. Increasing production without sacrificing quality is a balancing act that many researchers face. Factors to consider include:
- Yield: Optimizing the synthesis to produce a greater number of high-quality CNTs in a shorter time frame.
- Cost-Effectiveness: Identifying economical raw materials and methods that can help reduce the overall production cost.
- Process Control: Developing methods that allow for real-time monitoring and adjustments in large-scale operations can enhance consistency.
Achieving these goals is essential for the wider adoption of carbon nanotubes in commercial products, such as lightweight materials in aerospace or energy storage systems in batteries.
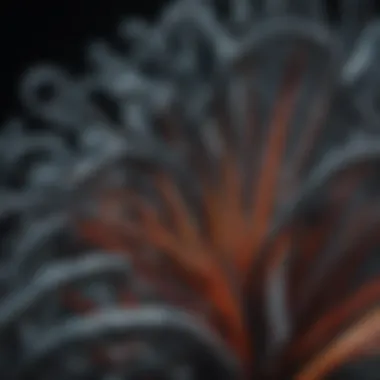
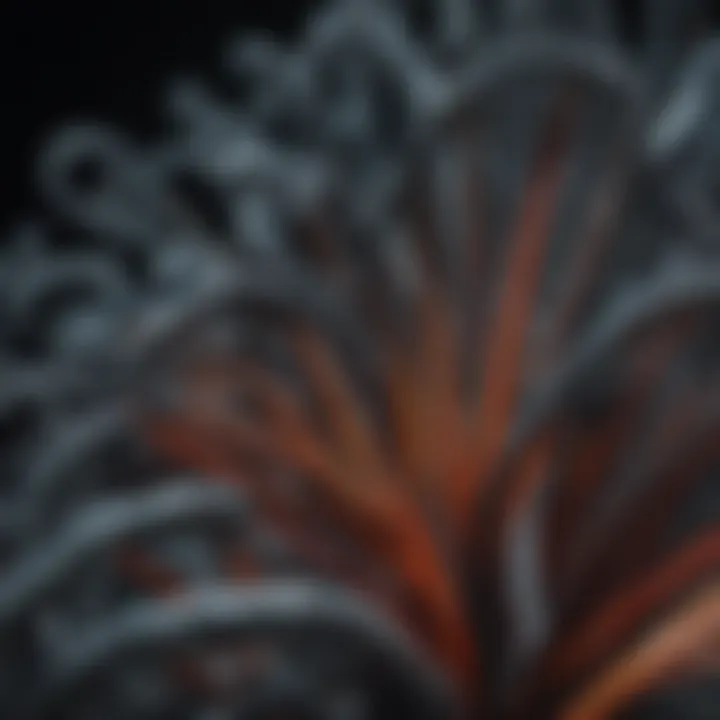
Health and Safety Concerns
Health and safety are significant considerations in the production and handling of carbon nanotubes. Some studies suggest that inhalation of CNTs may pose risks similar to asbestos fibers, which raises concerns about worker safety during manufacturing and research. There are several aspects to delve into:
- Exposure Risks: Workers in environments where carbon nanotubes are handled must be aware of potential inhalation and skin contact exposures. Effective personal protective equipment and ventilation systems must be implemented.
- Toxicity Studies: Research into the biological effects of carbon nanotubes is ongoing. Understanding how these materials interact with living organisms is crucial for establishing safety guidelines.
- Regulatory Frameworks: As the use of CNTs grows, regulatory bodies will need updated frameworks to monitor and control their use effectively. This includes safe disposal methods and guidelines for minimizing exposure.
Thus, addressing health and safety concerns is not just a legal obligation but a moral imperative to safeguard the well-being of workers and consumers alike.
Future Directions in CNT Research
Advanced research in carbon nanotubes (CNTs) holds significant promise in various fields of science and technology. The focus on future directions includes exploring novel applications and enhancing existing methods. This section provides a thorough overview of these potential advancements, highlighting their implications and relevance.
Nanocomposites
Nanocomposites integrate carbon nanotubes with other materials, resulting in enhanced characteristics. By including CNTs in polymers, ceramics, or metals, researchers aim for improved mechanical, thermal, and electrical properties. The primary benefit lies in the lightweight nature of nanocomposites, which can have substantial applications in aerospace and automotive industries. Furthermore, as the demand for superior materials increases, the role of CNTs in creating durable and efficient nanocomposites becomes even more critical.
Considerations for future studies in this area include the optimization of CNT distribution within matrices and the examination of interfacial bonding, which significantly affects the performance of the composites. The ability to tailor these nanocomposites for specific applications can lead to considerable advancements in technology and material science.
Energy Storage Applications
The potential of carbon nanotubes in energy storage technologies is another crucial area of research. Particularly, CNTs may enhance battery and supercapacitor performance. Their unique structure allows for higher surface area and improved charge storage. Utilizing CNTs can lead to batteries that charge faster and last longer, offering a compelling solution to current limitations in energy storage.
Energy-efficient technologies are vital in addressing global energy challenges. The integration of CNTs could result in systems with reduced size and weight, providing more space for energy-dense materials. Researchers continue to investigate the full range of CNTs' capabilities in energy storage, focusing on stability and efficiency.
Integration into Everyday Products
The integration of carbon nanotubes into everyday products represents an exciting trajectory for technology. From consumer electronics to sporting goods, CNTs can enhance performance and longevity. For example, CNT-based coatings can improve scratch resistance in screens and extending their life.
Research is also moving towards implementing CNTs in biomedical devices, where their conductive properties can facilitate better device interfaces and improved patient outcomes.
With the increasing trend towards smart materials, the adaptability of CNTs in everyday products leads to innovative applications that can change how we interact with technology.
"The future of carbon nanotubes lies not only in their unique properties but also in their ability to be integrated into a wide range of applications, making them one of the most versatile materials available today."
In summary, future directions in CNT research not only promise advances in materials science and engineering but also extend to practical applications that can reshape our everyday life. As the field evolves, new methods and collaborations across disciplines will be essential to harness the full potential of carbon nanotubes.
Ethical Considerations
In today's world, the discussion around the synthesis and application of carbon nanotubes (CNTs) extends beyond science and engineering. Ethical considerations are essential to ensure responsible innovation while harnessing the benefits of this advanced material. The implications of CNTs can affect numerous facets of society, from environmental sustainability to health impacts. Addressing these concerns is crucial for guiding future research and application practices.
Environmental Impact
The environmental impact of carbon nanotubes is a growing concern among researchers and industry leaders. The production processes associated with CNTs may lead to ecological disturbances if not managed properly. For instance, chemical vapor deposition, often used for creating CNTs, can release pollutants. The proper disposal of waste products and minimizing emissions are critical components of the overall sustainability strategy for CNT manufacturing.
Moreover, considering the life cycle of carbon nanotubes from their synthesis to end-of-life is vital. CNTs possess unique chemical stability, which may pose challenges in biodegradation and recycling. A comprehensive understanding of their environmental footprint can promote responsible management practices. Efforts must be made to analyze the long-term effects of CNTs on ecosystems, ensuring that their applications do not lead to unintended ecological consequences.
Sustainability in Production
Sustainability is another cornerstone in the ethical discourse surrounding carbon nanotubes. As the demand for these materials rises, production methods must adapt to ensure minimal resource consumption and environmental degradation. Techniques that are energy-intensive or generate substantial waste require reevaluation.
Research into greener production methods, such as utilizing renewable energy sources for synthesis, should be a priority. Additionally, the development of recycling strategies for CNTs will contribute to a circular economy, thus mitigating waste. Achieving sustainability in CNT production not only aligns with ethical practices but also promotes innovation and economic viability.
"The responsible use of carbon nanotubes will define their legacy in modern technology."
In summary, ethical considerations in the synthesis and application of carbon nanotubes encompass environmental impacts and sustainable production practices. By prioritizing these elements, the scientific community can ensure that the advancements in CNT technology yield benefits for society while minimizing harm.
Epilogue
The conclusion serves as the final reflection on the complex and multifaceted subject of carbon nanotubes (CNTs). In this section, we synthesize the main points discussed throughout the article and articulate their significance in both current and future contexts. The importance of CNTs lies in their extraordinary properties, including exceptional electrical conductivity, mechanical strength, and thermal properties. These attributes allow for innovative applications across various fields, from electronics to medicine.
One of the key elements covered includes the various synthesis methods such as chemical vapor deposition and arc discharge. Each method presents its own advantages and challenges, influencing the quality and applicability of the produced carbon nanotubes. Understanding these methods is critical for researchers aiming to advance the use of CNTs in practical applications.
Additionally, ethical considerations surrounding the production and application of CNTs cannot be overlooked. Issues like environmental impact and sustainability are paramount in the discourse on nanotechnology. Researchers and companies involved in CNT production must prioritize these factors to ensure a responsible approach to embracing this advanced material.
Moreover, this article emphasizes the future directions in CNT research. The development of nanocomposites and energy storage solutions are areas of growth that promise to enhance the integration of carbon nanotubes into everyday products. This presents many opportunities for innovation and advancement in technology.
In summary, our exploration of carbon nanotubes is not merely academic. It provides a foundation for understanding a material that is poised to influence numerous industries profoundly. The implications of advancements in CNTs affect not only scientific communities but also the general public and future generations. Therefore, grasping the intricacies of their synthesis, properties, and applications remains of utmost importance.
Summary of Key Points
- Carbon Nanotubes Properties: They exhibit remarkable electrical, thermal, and mechanical properties, crucial for a range of applications.
- Synthesis Methods: Chemical vapor deposition, laser ablation, and arc discharge are key methods, each with unique advantages and challenges.
- Applications: CNTs are relevant in electronics, materials science, medicine, and environmental technology.
- Ethical Considerations: Sustainability and environmental impact must be addressed in the production and use of CNTs.
- Future Research Directions: Exploration of nanocomposites and energy storage solutions highlights the ongoing potential of CNTs in modern technology.