Understanding Solar Panel Temperature Ranges Effectively
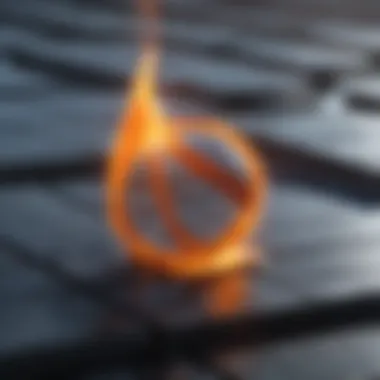
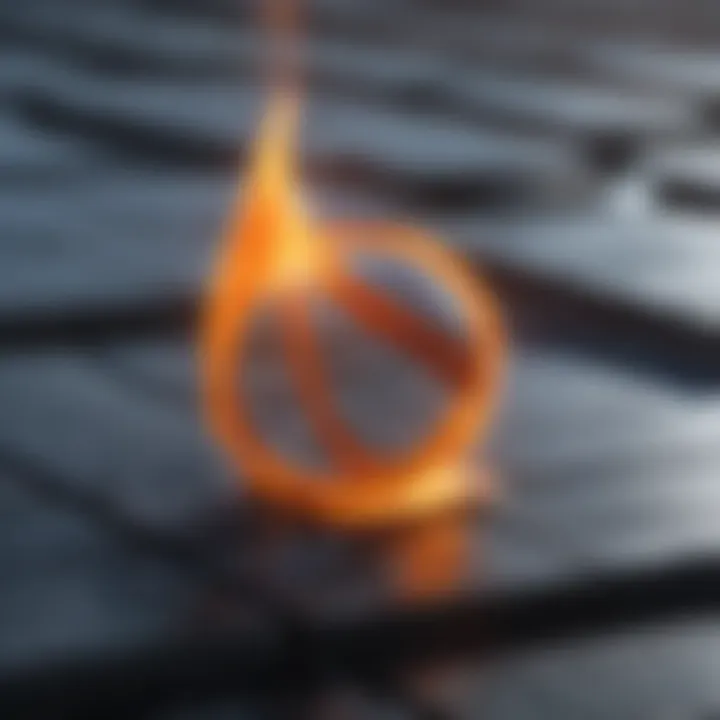
Intro
Solar panels play a crucial role in the global shift toward renewable energy. However, their efficiency is significantly influenced by temperature. Understanding how solar panels operate within specific temperature ranges is essential for optimizing their performance and longevity. This article provides a detailed exploration of the relationship between temperature and solar panel functionality, the materials involved in their creation, and effective strategies to maximize energy generation in varying conditions.
Key Concepts and Terminology
Definition of Key Terms
- Solar Panels
These are devices that convert sunlight into electricity using photovoltaic cells. They are essential components of solar energy systems. - Temperature Coefficient
A measure that indicates how much a solar panel's output decreases with an increase in temperature. It is expressed as a percentage per degree Celsius. - Maximum Power Point Tracking (MPPT)
A technique used in solar inverters to optimize the energy output by adjusting the electrical load on the panel.
Concepts Explored in the Article
- Impact of Temperature on Efficiency
The article discusses how temperature affects solar efficiency. Higher temperatures can reduce the voltage output of solar panels, leading to decreased overall performance. - Material Science
Examination of the materials used in solar panels and how they interact with temperature fluctuations. Different materials have varying thermal properties which can influence their performance. - Optimization Strategies
Practical approaches to mitigate the adverse effects of temperature on solar panel efficiency will be analyzed. This includes installation techniques and maintenance practices.
Overview of Solar Panel Functionality
The functionality of solar panels is essential to comprehend for anyone engaged in solar energy systems. These panels convert sunlight into electricity, which leads to a sustainable energy source. Understanding how they work aids in optimizing performance, especially in different temperature ranges, as heat can significantly influence energy efficiency. The operation of solar panels must be analyzed through basic principles, efficiency factors, and the impact of environmental conditions.
Basic Principles of Solar Power Generation
Solar panels mainly rely on photovoltaic technology. The primary concept involves semiconductors, commonly silicon, which excite electrons upon exposure to sunlight. This excitement causes the electrons to flow, generating direct current (DC) electricity. The flow of electricity is then converted into alternating current (AC) through an inverter.
The efficiency of this energy conversion process is dependent on several factors:
- Sunlight Intensity: More sunlight results in higher electricity production.
- Panel Angle: The angle of the panels relative to the sunlight increases or decreases exposure.
- Temperature: Finally, operational temperatures also play a crucial role. Higher temperatures can reduce efficiency due to increased resistance in the panels.
Role of Temperature in Energy Conversion
Temperature is a critical factor affecting solar panel functionality. Every solar panel has an optimal operating temperature, often between 15°C and 35°C. When temperatures rise above this range, there is a shift in efficiency. Indeed, high temperatures can lead to a decline in output.
In a high-temperature environment, the increase in electron movement can result in decreased energy conversion efficiency, as the increased thermal energy can impede the organized flow of electrons. Thus, it is vital to monitor the temperature surrounding the panels.
"Solar panels perform optimally at lower temperatures, contrary to common assumptions about how heat may enhance energy generation."
Recognizing the balance between temperature and performance ensures better energy yield and increases the lifespan of solar panel systems. Understanding these principles forms the groundwork for examining the influence of temperature and how to mitigate adverse effects in solar energy systems.
Temperature Ranges for Solar Panels
Temperature plays a significant role in determining the efficiency and performance of solar panels. Understanding the temperature ranges within which these panels operate optimally is crucial for maximizing energy generation. This section delves into optimal operating temperatures and the impact of extreme temperatures on solar panel functionality. By grasping these concepts, stakeholders can implement strategies that enhance the overall efficiency and lifespan of solar energy systems.
Optimal Operating Temperature
Solar panels are designed to work effectively within a specific temperature range. Typically, the optimal operating temperature for most photovoltaic systems lies between 15 and 35 degrees Celsius. At temperatures within this range, solar panels can convert sunlight into electricity with greater efficiency.
When temperatures exceed this ideal range, the efficiency of solar panels often diminishes. The temperature coefficient indicates how much efficiency drops with increasing temperature. For instance, a typical silicon solar panel might have a temperature coefficient of -0.4% per degree Celsius. This means that for every degree above 25 degrees Celsius, the panel’s output can decrease by approximately 0.4%.
Therefore, to maintain optimal performance, it is vital to monitor environmental conditions closely. Placing solar panels in areas where they can receive shade during peak sun hours can be an effective strategy. Additionally, using mounting systems that allow for airflow can help mitigate heat buildup.
Extreme Temperatures: Effects on Performance
Extreme temperatures can significantly affect solar panel performance in negative ways. When exposed to extremely high temperatures, the risk of reduced efficiency increases, leading to lower energy output. Conversely, extremely low temperatures might not directly harm the performance but can affect the underlying components, such as inverters.
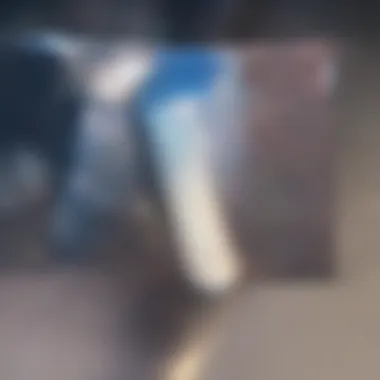
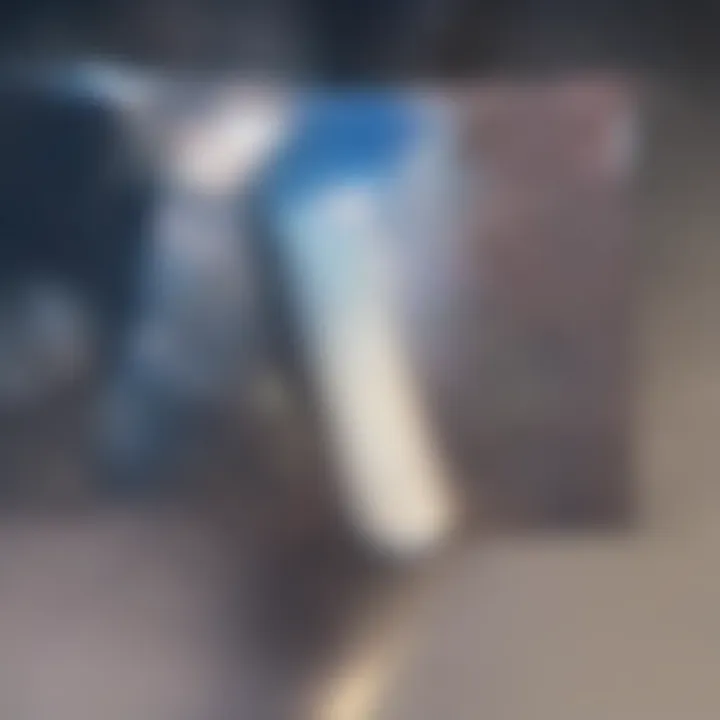
Important insight: High ambient temperatures can not only lower energy output but also potentially damage the panels in the long term, leading to additional maintenance costs.
Several studies have shown that solar panels can sustain structural integrity at various temperatures. However, prolonged exposure to high temperatures can accelerate material degradation. For example, components such as the backsheet, glue, and silicon cells may break down faster under such stress.
In colder climates, while solar panels may function well, conditions like snow accumulation or ice can create challenges for energy generation. It is important to ensure that primary installation areas have appropriate tilt angles to prevent snow buildup.
In summary, understanding both the optimal operating temperature and the repercussions of extreme temperatures is essential for improving the performance and longevity of solar panels. Future efforts may focus on enhancing material properties to withstand temperature fluctuations more effectively.
Construction Materials and Temperature Sensitivity
Understanding construction materials used in solar panels is crucial for assessing their performance under varying temperature conditions. Different materials respond uniquely to thermal changes, impacting both efficiency and longevity of solar systems. The choice of these materials directly determines how well the panels can ventilate heat and manage extreme temperatures. This section examines the key types of solar panels and their materials, as well as the implications of temperature sensitivity on energy generation.
Silicon-Based Solar Panels
Silicon-based solar panels are the most commonly used type in the industry. They are made from crystalline silicon, a material that is quite effective in converting sunlight into electricity. Silicon has a positive characteristic: it maintains its efficiency across a range of temperatures. However, performance can decline if temperatures rise above the optimal threshold of approximately 25 degrees Celsius. When exposed to higher temperatures, silicon's bandgap can change, leading to lesser energy production.
To mitigate this, manufacturers often incorporate anti-reflective coatings and back sheets, which can help reduce heat absorption. The efficiency rating of these panels commonly drops by about 0.5% for every degree Celsius increase above this optimal temperature. Therefore, understanding how silicon reacts to temperature fluctuation can guide better system design and operational strategies.
Thin-Film Solar Panels
Thin-film solar panels offer a different approach, utilizing materials like cadmium telluride or amorphous silicon. These panels are lighter and can be more flexible than traditional silicon panels. A significant benefit of thin-film panels is their lower temperature sensitivity. They can perform slightly better at elevated temperatures compared to silicon panels. The performance drop for thin-film panels is less severe; it's about 0.2% per degree Celsius increase.
However, thin-film technology comes with trade-offs. Their efficiency is generally lower than that of crystalline silicon panels, which means larger surface areas are required to generate the same amount of power. This aspect can become a limiting factor in space-constrained installations. The materials in thin-film panels can also degrade under prolonged exposure to som factors, including humidity and temperature cycling.
Impact of Temperature on Material Integrity
The integrity of the materials used in solar panels is paramount for long-term durability. High temperatures can lead to physical changes in materials. For example, prolonged exposure to extreme heat may result in delamination of layers in the solar panel structure, causing a decline in efficiency.
When evaluating the temperature sensitivity of solar panels, one must consider:
- Thermal expansion: Materials expand and contract as temperature changes, potentially leading to micro-cracks.
- Degradation: UV radiation can exacerbate material breakdown under high temperature.
- Sealant performance: Sealants used in the construction must withstand thermal variations without failing, ensuring the longevity of the panel.
The interaction between temperature fluctuations and material integrity is critical. Poorly designed panels may experience significant efficiency losses or ultimately fail if not adequately managed.
Temperature Coefficient and Its Implications
The temperature coefficient is a critical parameter when evaluating the performance of solar panels. It quantifies how much the output of a solar panel changes as the temperature fluctuates. Understanding this coefficient allows users to optimize their solar systems effectively. The implications of the temperature coefficient impact efficiency, design considerations, and economic viability, making it essential for anyone involved in solar energy.
Understanding Temperature Coefficient
The temperature coefficient represents the percentage decrease in power output for each degree Celsius increase in temperature. For instance, a common temperature coefficient for silicon-based panels is -0.4% per °C. This means that for every degree above 25°C, the efficiency of the panel drops by 0.4%. This metric is vital, as higher temperatures can lead to significant losses in energy production.
Factors influencing this coefficient include the type of materials used in the solar panel, their design, and the location where they are installed. Higher quality materials tend to have lower negative coefficients, preserving more efficiency.
Calculating Efficiency Loss
Determining the efficiency loss due to temperature requires straightforward calculations. First, determine the baseline efficiency of the solar panel at a standard temperature, typically 25°C. Then, use the following formula:
[ \textEfficiency Loss = \textTemperature Coefficient \times (T - 25) \times \textBase Efficiency ]
Where:
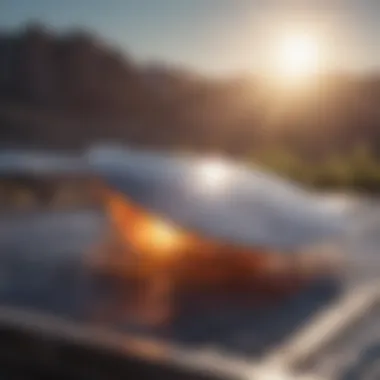
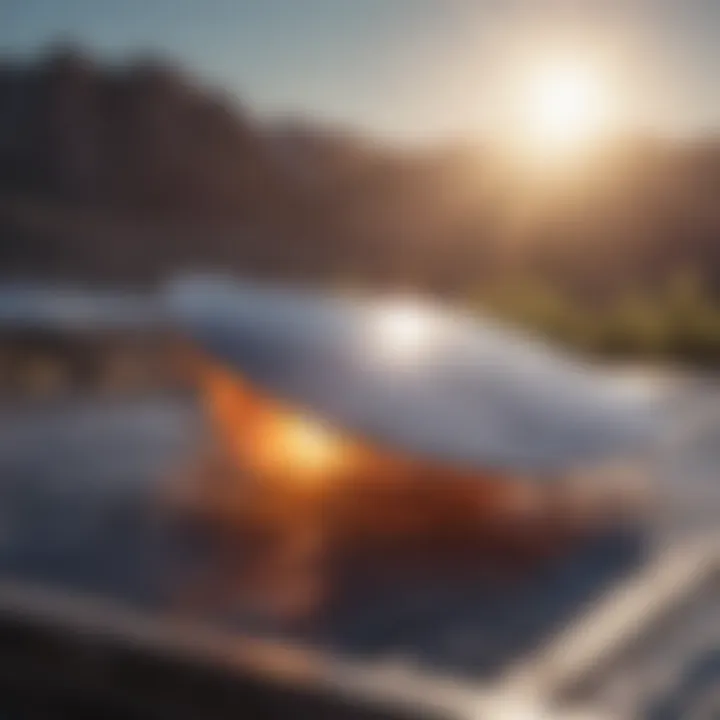
- Efficiency Loss is the reduction in performance
- Temperature Coefficient is expressed in decimal form
- T is the actual temperature in °C
- Base Efficiency is the efficiency at 25°C
For example, if a solar panel has a base efficiency of 18% and a temperature coefficient of -0.4% per °C, at 35°C, the calculation would be:
Hence, at 35°C, the solar panel will lose approximately 0.72% of efficiency due to temperature increase. Understanding this loss and how to manage it effectively can lead to substantial improvements in energy production.
“[] Knowledge of the temperature coefficient not only aids in forecasting energy output but also is invaluable in selecting the right solar technology for different climates and conditions.”
Environmental Factors Influencing Temperature Range
Understanding the environmental factors that influence the temperature range of solar panels is crucial for optimizing their performance. These factors can significantly affect both energy generation and long-term durability. By comprehending how environmental conditions impact solar panel operation, stakeholders can make informed decisions regarding installation, maintenance, and usage.
Geographical Considerations
Geographical location plays a significant role in determining the temperature range experienced by solar panels. For instance, panels installed in desert regions may face extreme heat, while those located in colder climates may experience lower temperatures. Key geographical considerations include:
- Altitude: Higher altitudes typically have cooler temperatures and can also affect panel efficiency due to thinner atmosphere.
- Latitude: Solar panels closer to the equator encounter more intense sunlight and higher average temperatures, impacting performance differently than panels at higher latitudes.
- Proximity to water bodies: Nearby oceans or lakes can moderate temperature fluctuations, providing a more stable climate for solar panels.
These geographical factors influence not only the operational efficiency of solar panels but also their durability over time.
Seasonal Variations
Seasonal changes can create a variety of temperature conditions for solar panels, and this variability must be accounted for in system design. Seasonal variations include:
- Temperature fluctuations: Panels may experience significant temperature swings from summer to winter. This change can lead to thermal expansion and contraction, potentially impacting the material integrity.
- Sunlight angle: The angle of solar radiation changes with the seasons, which can impact how much energy solar panels are able to generate despite temperature.
- Weather patterns: Rain, snow, and cloud cover can also affect panel performance. For instance, while snow can insulate panels from extreme cold, it may also block sunlight.
Each season brings unique challenges and opportunities for solar energy production, and understanding these can help in optimizing the installation.
Impact of Climate Change
Climate change introduces additional complexity to the temperature dynamics of solar panels. As global temperatures continue to rise, the implications for solar energy systems become more pronounced:
- Increased temperatures: Higher average temperatures can lead to a decrease in panel efficiency. This underscores the importance of managing heat dissipation effectively.
- Extreme weather: Increased incidence of extreme weather events, such as hurricanes, floods, or prolonged droughts, can damage solar panel systems and reduce overall energy generation.
- Shifts in solar radiation: Changes in atmospheric conditions may affect the amount and quality of solar radiation available, directly impacting performance.
Understanding these implications is essential for stakeholders in the solar industry as they position their investments for the future.
Harnessing solar energy efficiently will increasingly depend on recognizing and mitigating the effects of environmental factors. By addressing geographical considerations, seasonal variations, and climate change impacts, one can enhance the viability and sustainability of solar energy systems across diverse settings.
Strategies for Temperature Management
Managing the temperature of solar panels is crucial for maintaining their efficiency and longevity. As temperature impacts the electrical output of solar panels, effective temperature management strategies can significantly enhance performance. This section discusses various methods to achieve optimal thermal conditions for solar energy systems, providing a comprehensive understanding for students, researchers, educators, and professionals.
Cooling Methods for Solar Panels
Cooling methods are essential for mitigating heat buildup in solar panels. Excessive heat can lead to deterioration in performance, reducing energy conversion efficiency. There are several effective cooling techniques employed in the industry:
- Passive Cooling: This includes natural airflow and designs that allow heat to dissipate without active intervention, such as ventilation through the mounting system.
- Active Cooling: Techniques that involve mechanical systems, such as fans or water pumps, to actively draw heat away from the panels. These systems can be more effective but require energy input, which must be accounted for in efficiency calculations.
- Reflective Coatings: Applying special reflective surface treatments can reduce the amount of heat absorbed by the solar panels, subsequently lowering their operating temperature.
Effective cooling methods can prolong the lifespan of solar panels by preventing heat-related damage and performance loss.
Optimal Orientation and Installation
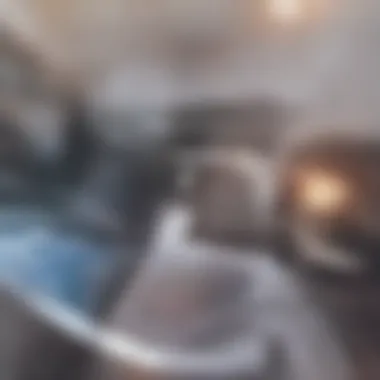
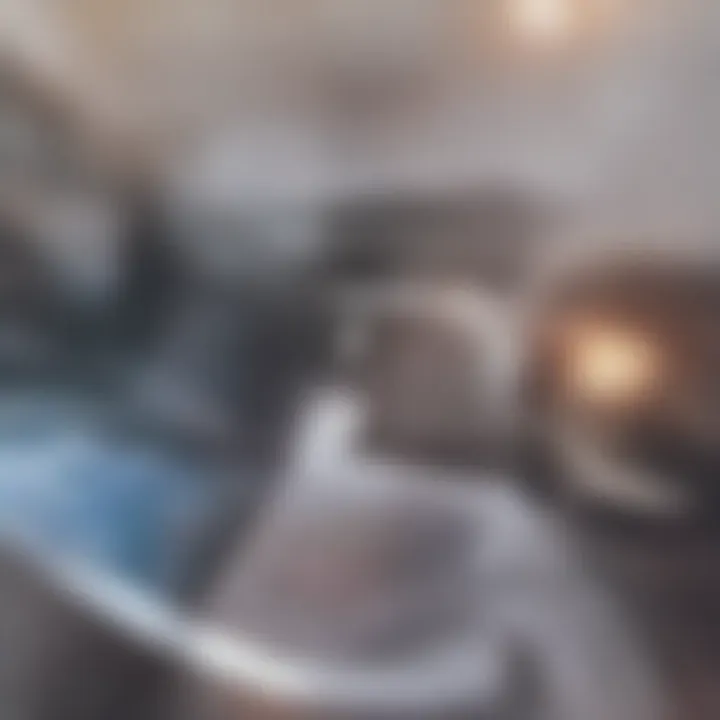
The placement and orientation of solar panels significantly influence their temperature management. Proper installation is key for maximizing both energy production and heat dissipation. Important considerations include:
- Angle of Installation: Panels tilted at the right angle can enhance sun exposure while allowing better heat escape. Experimenting with different angles based on regional sunlight patterns can lead to improvements in performance.
- Spacing Between Panels: Adequate space allows for airflow around individual panels, enhancing cooling effects. Crowded installations trap heat and can lead to less efficient systems.
- Avoiding Heat Sources: Installing solar panels away from heat-generating structures or surfaces can prevent additional heat stress on the panels, aiding in performance optimization.
Innovative Technologies for Thermal Regulation
Recent advancements in technology have led to innovative solutions for thermal regulation in solar panels. These technologies enhance energy efficiency by minimizing the effects of temperature variations. Some notable developments include:
- Phase Change Materials (PCMs): These materials absorb heat during the day and release it when temperatures drop, helping to stabilize panel temperatures.
- Thermal Sensors: Integrated monitoring systems can detect excessive heat and activate cooling methods when needed, ensuring that panels maintain optimal operating conditions.
- Bifacial Panels: These panels can capture sunlight from both sides and are often designed to operate at lower temperatures due to the increased cooling effect from the rear surface.
Economic Implications of Temperature Effects
Understanding the economic implications of temperature effects on solar panels is essential. As the performance of these systems is highly dependent on temperature, any variabilities in this regard can directly influence financial outcomes. When solar panels operate outside their optimal temperature range, their efficiency drops. This means lower energy production, which ultimately affects the return on investment for solar panel systems. Therefore, recognizing and managing temperature-related impacts is critical for maximizing economic benefits in solar energy harvesting.
Cost Analysis of Temperature Management
Effective temperature management can mitigate performance losses in solar panels. The cost analysis involves evaluating investment in cooling systems or other technologies designed to maintain optimal temperatures. Here are key points to consider:
- Initial Costs: Installing advanced cooling technologies or reflective coatings may require a significant upfront investment. However, this must be weighed against the potential loss in efficiency due to excessive heat.
- Operational Costs: Some cooling methods might incur ongoing expenses, such as energy consumption for mechanical fans or pumps.
- Maintenance Costs: Regular maintenance is necessary for any advanced cooling mechanism to operate efficiently.
The figure below outlines typical expenses involved in temperature management:
| Expense Type | Estimated Cost | | Initial Setup | $5,000 - $20,000 | | Annual Maintenance | $500 - $2,000 | | Operational Costs | Variable |
While it might seem like a considerable investment, the savings from improved energy efficiency can lead to a quicker return on investment.
Long-term Financial Benefits of Efficient Systems
Long-term financial benefits from efficient solar systems can significantly outweigh initial investments in temperature management. Benefits may include:
- Increased Energy Production: By ensuring panels operate in their ideal temperature range, energy yield maximizes, directly increasing revenue from power sales.
- Improved System Longevity: Properly managed systems often have extended operational lifespans, reducing replacement costs over time.
- Potential Incentives: Governments may offer financial incentives or tax rebates for implementing energy-efficient technologies.
"A well-managed solar system not only leads to higher efficiencies but helps in sustaining lower costs over its lifetime."
In summary, investing in temperature management strategies pays off in the long run. These improvements not only benefit the immediate financial landscape but also contribute to broader energy sustainability goals. Evaluating both short-term costs and long-term benefits is vital for stakeholders in the solar sector to make informed decisions.
Epilogue
In this article, we have explored various facets of how temperature impacts solar panel performance and durability. Understanding the temperature range in which solar panels function optimally is crucial for maximizing their efficiency and lifespan. This conclusion aims to synthesize key insights and highlight the essential considerations outlined in our discussion.
Summarizing Key Insights
Solar panels are designed to convert sunlight into energy, but various environmental factors can significantly influence their efficiency. The optimal operating temperature is usually between 15°C and 25°C. Within this range, solar panels exhibit maximum energy conversion. However, as temperatures rise above this range, energy output tends to decline, a phenomenon driven by the temperature coefficient of the materials used.
Key points include:
- Temperature Coefficient: Understanding how efficiency drops with increasing temperature is vital for evaluating solar panel performance in specific climates.
- Material Impact: Silicon-based and thin-film solar panels respond differently to temperature fluctuations, affecting their reliability.
- Cooling Strategies: Implementing cooling methods can dramatically enhance energy generation, particularly in hotter regions.
These insights underscore the importance of considering temperature when designing and installing solar energy systems.
Future Directions in Solar Research
As climate change continues to alter environmental conditions, future research must focus on innovative solutions for optimizing solar panel performance across varying temperature scenarios. Key areas of exploration include:
- Advanced Materials: Research into new materials can improve temperature resilience, allowing solar panels to maintain performance even in extreme conditions.
- Thermal Management Technologies: Developing systems that dynamically adjust to temperature changes will be essential for enhancing efficiency.
- Data Analytics: Using big data and machine learning can help in predicting optimal setup conditions based on historical temperature data.
As we progress towards more sustainable energy solutions, the interplay between solar technology and temperature management remains a pivotal area of study.